All published articles of this journal are available on ScienceDirect.
Epigenetic Modifications in Obesity and Type 2 Diabetes
Abstract
Obesity is a chronic condition that is also a risk factor of several other chronic conditions including type 2 diabetes. The effects of maternal obesity and type 2 diabetes on fetal development and offspring health are mediated through the transmission of epigenetic modifications in addition to the possible permanent changes of the organs caused by the intrauterine environment hypothesized by the Developmental Origins of Health and Disease (DOHaD) theory. Epigenetic modifications can be altered by environmental factors including dietary and lifestyle factors. The current priorities include identification and confirmation of the specific epigenetic biomarkers associated with obesity and type 2 diabetes in human subjects and identification of the dietary and lifestyle factors that contribute to each of the identified specific epigenetic biomarkers.
1. INTRODUCTION
The prevalence of obesity and type 2 diabetes has dramatically increased in the past 3 decades [1]. Obesity is defined by increased body weight, body mass index (BMI) equal or more than 30 kg/m2, as a result of the accumulation of extra energy as lipids in the adipose tissues. Obesity is associated with insulin resistance and low-grade inflammation [2]. Type 2 diabetes is characterized by insulin resistance associated with dysregulation of blood glucose homeostasis [3]. Therefore, obesity and type 2 diabetes are closely related chronic conditions with some shared common characteristics, including higher blood glucose levels caused by insulin resistance. There are more than 90% of type 2 diabetes patients developed from obesity [3]. On the other hand, children of the mothers with diabetes have increased risk to become obese [4]. Obesity is also a risk factor for other chronic conditions, including cardiovascular disease, hypertension, cancer, etc [5, 6]. The etiology studies reveal that obesity and type 2 diabetes are caused by genetic and environmental factors, including dietary and lifestyle factors [7]. However, the pathologic mechanisms involved in the development of obesity and type 2 diabetes are still not totally clear.
2. THE MECHANISMS OF DEVELOPMENT OF OBESITY
It has been speculated by the Developmental Origins of Health and Disease (DOHaD) hypothesis that the fetal and infant phases of life have effects on the development of adult obesity and metabolic disorders [8]. The fetus makes predictive adaptations in response to the factors in the intrauterine environment, resulting in permanent adjustments in organs involved in the homeostatic regulations to adapt to the intrauterine and possible adverse postnatal environment [9]. These permanent changes of certain organs at specific developmental stages of the fetus caused by the intrauterine environment factors may have adverse long-term health effects on the adult offspring. This hypothesis can be perfectly used to explain that the children born to the mother exposed to famine during the pregnancy had increased adiposity and metabolic disorders [9]. However, organisms survive by adapting to the constantly changing environment over time not only at one time point. Recent studies indicate that epigenetic modifications altered by environmental factors, including dietary and lifestyle factors, are involved in the development of obesity and type 2 diabetes, in addition to the involvement of genetic factors such as single nucleotide polymorphisms (SNPs) [10-14]. Epigenetic modifications, the modifications on top of the genetic coding, are the modifications added to the genome rather than changing the genetic coding. These epigenetic modifications typically include genomic DNA methylation, histone tail modifications, and non-coding small RNAs (ncRNAs) such as microRNAs (miRNAs) [7, 15]. It has been demonstrated that these epigenetic modifications can affect each other. For example, methyl CpG-binding protein 2 binds to methylated DNA and subsequently recruits enzymes such as histone deacetylases and histone demethylases to modify histones [16-18]. Environmental factors including dietary and lifestyle factors induce changed levels of epigenetic modifications and subsequently cause a change of expression of genes involved in the development of chronic diseases [7, 11, 19]. In real life, it takes long periods of time for the environmental factors to cause changes of epigenetic modifications involved in development of chronic conditions. That is why chronic conditions such as obesity, type 2 diabetes, and cardiovascular disease may take years and decades to develop.
3. TRANSMISSION OF EPIGENETIC MODIFICATIONS OF OBESITY TO THE OFFSPRING
The statistical data from the Centers for Disease Control and Prevention (CDC) indicate that increasing prevalence of childhood obesity is well correlated with increasing adulthood obesity and type 2 diabetes [1, 20]. Human obesity and diabetes are associated with reproductive disorders, including low fertility [21]. It is interesting to notice that the maternal effects are considered in most of the human studies addressing childhood obesity, while the sperms from the paternal side are being paid more attention when addressing reproductive issues of parents with obesity and type 2 diabetes [8, 20, 21]. In addition, it has been demonstrated using rodent models that both maternal oocytes and paternal sperms with altered epigenetic modifications from obese parents are involved in affecting the health of the offspring adversely [22-24]. These lines of evidence suggest that altered epigenetic modifications of gametes from obese parents are involved in passing on obesity to the offspring. The detailed mechanisms of transgenerational transmission of epigenetic modifications associated with obesity are being addressed at the current time.
3.1. Effects of Maternal Epigenetic Modifications of Obesity
The effects of maternal obesity and type 2 diabetes on fetal development and offspring health are mediated through the epigenetic modifications transmitted from the mother to the offspring, in addition to the possible permanent changes of the organs caused by the intrauterine environment hypothesized by the DOHaD theory [25]. Increased overall DNA methylation levels are observed in oocytes of mice of both high-fat diet and ob/ob obese mouse models with increased methylation levels of the Leptin gene promoter and decreased methylation of the promoter of PPARα gene that encodes for a transcription factor mediating adipogenesis [22, 26]. In the adipose tissues of diet induced obese mice, DNA methylation levels of the Leptin gene promoter and the binding of DNA methyltransferases (DNMTs) are increased [27]. Differential methylation patterns are observed in oocytes of type 1 diabetes mouse model and bovine blastocytes under in vitro hyperinsulinemia [28, 29]. In mouse models, maternal diabetes affects methylation levels of imprinted genes in oocytes associated with decreased expression of DNMTs [21]. The studies on modifications of protein molecules in obesity and diabetes, such as acetylation of superoxide dismutase (SOD), that are not affecting gene expression should not be considered epigenetic modifications, even deacetylation of SOD plays an important protective role against oxidative stress in oocytes [21].
3.2. Effects of Paternal Epigenetic Modifications of Obesity
Research on transgenerational transmission indicates that obese male individuals affect the health of the offspring through the epigenetic modifications carried by the sperm, including altered levels of global DNA methylation, histone acetylation, and ncRNA [24, 30, 31]. Male obesity model induced by high-fat diet affects the metabolism and reproduction of the F1 generation associated with reduced sperm motility and increased DNA damage [32]. High-fat diet reduces global genomic DNA methylation levels in the sperm and in the spermatozoa of the offspring [33, 34]. Increased H3K9 acetylation associated with decreased sirtuin 6 (SIRT6) deacetylase in the nucleus of male mouse sperm caused by high-fat diet is associated with increased DNA damage [35]. These results further confirmed that increased overall histone acetylation levels during sperm maturation caused by inhibition of histone deacetylase by Trichostatin A are associated with decreased sperm quantity and DNA damage [36, 37]. When high fat diet induced obesity mouse model was used to study the alteration of the sperm miRNA levels, differential expression of miRNAs was observed in the sperms of the obese mice induced by high fat diet, however, the same set of the abundant miRNAs was not observed in the sperms of the F1 offspring male mice even the metabolic and reproductive phenotypes were also observed on the F2 offspring mice [31]. This evidence suggests that there are other mechanisms in addition to epigenetics involved in transgenerational transmission of obesity. DOHaD hypothesis might be one of the possible mechanisms.
4. QUESTIONS AND PERSPECTIVES
As epigenetic modifications can be altered by environmental factors, including dietary and lifestyle factors, the mechanisms remain unclear on how malnutrition in early life, including energy imbalance, can have long-term effects such as obesity and metabolic disorders on the adult offspring. It is likely that epigenetic modifications from the gametes and the permanent adjustments in fetal organs caused by the intrauterine environment factors are both involved in affecting the health of the offspring (Fig. 1). As epigenetic modifications such as DNA methylation affect genetic alterations, the environment also shapes the species through epigenetic modifications during the selection process [38]. Since obesity is a chronic condition that is mostly related to dietary and lifestyle factors as the typical environmental factors, it is expected that many of the remaining questions related to the role of epigenetic modifications in the development of chronic diseases will be first addressed in obesity and type 2 diabetes. The knowledge of epigenetics in obesity and type 2 diabetes will accelerate the studies to address the involvement of epigenetic modifications caused by environmental factors in the development of other chronic conditions.
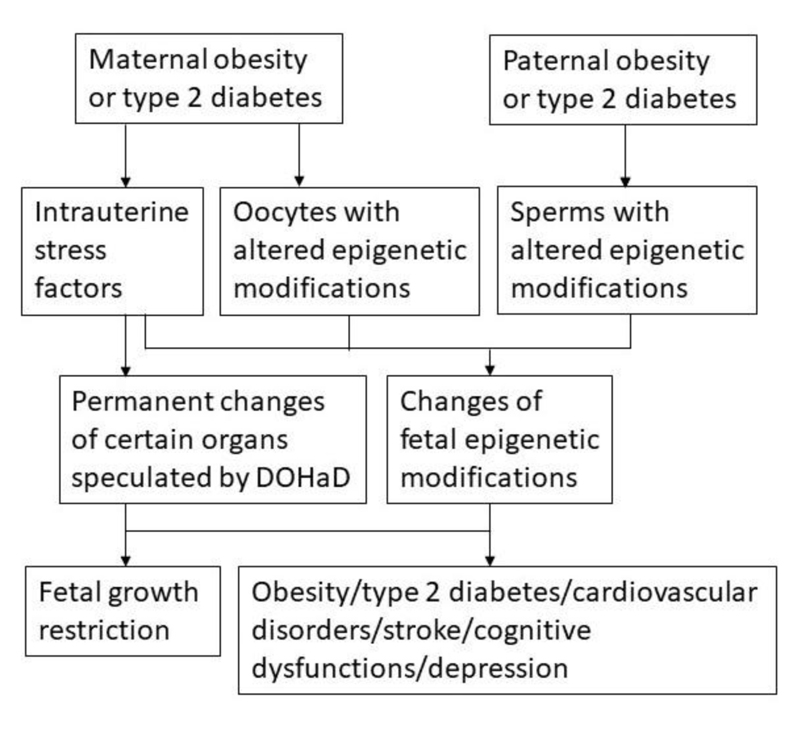
CONCLUSION
It is a priority at this time to identify and confirm the specific epigenetic biomarkers associated with obesity and type 2 diabetes in human subjects. These biomarkers can be used to develop prognosis strategies. Another priority is to identify the dietary and lifestyle factors that contribute to each of the identified specific epigenetic biomarkers. This knowledge will facilitate developing management strategies for prevention and treatments. Some of the miRNA epigenetic biomarkers associated with obesity and type 2 diabetes may be used directly to develop drugs. For example, if a specific miRNA is identified to facilitate changing white adipocytes to become brown adipocytes, then this miRNA can be synthesized and delivered into white adipose tissues to treat obesity.
LIST OF ABBREVIATIONS
DOHaD | = Developmental Origins of Health and Disease |
BMI | = Body Mass Index |
CDC | = Centers for Disease Control and Prevention |
SOD | = Superoxide Dismutase |
CONSENT FOR PUBLICATION
Not applicable.
FUNDING
This work was supported by startup funds from Saint Louis University to YL.
CONFLICT OF INTEREST
The author declares no conflict of interest, financial or otherwise.
ACKNOWLEDGEMENT
Declared none.