Protein Kinase A Subunit α Catalytic and A Kinase Anchoring Protein 79 in Human Placental Mitochondria
Abstract
Components of protein phosphorylation signalling systems have been discovered in mitochondria and it has been proposed that these molecules modulate processes including oxidative phosphorylation, apoptosis and steroidogene-sis.
We used electrophoresis and Western blots probed with specific antibodies to protein kinase A a catalytic subunit (PKAα Cat) and A kinase anchoring protein of approximately 79 kDa molecular weight (AKAP79) to demonstrate the presence of these two proteins in human placental mitochondria. Heavy mitochondria characteristic of cytotrophoblast were sepa-rated from light mitochondria characteristic of syncytiotrophoblast by centrifugation. PKAα Cat and AKAP79 were pre-sent in both heavy and light mitochondria with no significant difference in concentration. Sucrose density gradient separa-tion of submitochondrial fractions indicated PKAα Cat is located predominantly in the outer membrane whereas AKAP79 is present mainly in the contact site fractions.
These data indicate that PKAα Cat is present in the cytoplasm, nucleus and mitochondria of placental cells. AKAP79 is also present in human placental mitochondria but there may be anchoring proteins other than AKAP79 responsible for fix-ing PKA to the outer membrane. PKA may play roles in mitochondrial protein phosphorylation systems in both cytotro-phoblast and syncytiotrophoblast.
INTRODUCTION
It has been known for some time that protein phosphorylation by protein kinases is an important component of cellular signalling systems that are located in the plasma membrane, nucleus and cytoplasm [1]. Relatively little is known, however, about cellular signalling from these regions to the mitochondria but several groups have explored the hypothesis that protein phosphorylation plays an important role in the signalling systems that operate in the mitochondrion [2-6]. In many tissues it is known that protein kinases phosphorylate and activate key proteins such as receptors, enzymes and ion channels and these can in turn then affect cellular functions such as metabolism, differentiation and endocrine functions [1, 2]. One of the most common protein kinases, cAMP-dependent protein kinase (PKA) can migrate to many areas of the cell and is often held in a precise cellular location by A kinase anchoring proteins (AKAPs) [7]. There are several forms of AKAP (often differentiated by a suffix corresponding to their molecular weight) that have been found in tissues and species other than placenta and human. The only form of AKAP found in human reproductive tissue prior to this investigation was AKAP79 which, was shown to be present in the cytoplasm of humanmyometrium and is thought to anchor PKA to the myometrial plasma membrane [8].
PKA is activated when cAMP binds to the two regulatory (Reg) subunits, which disengage to release consequently active catalytic (Cat) subunits. There are three known isoforms of the Cat subunit; these are a Cat, b Cat, and ã Cat and are four known isoforms of the Reg subunit, these are type Ia Reg, Ib Reg, IIa Reg and IIb Reg [9]. PKA is only active when cAMP binds to the Reg subunits, causing Cat subunits to dissociate as free and active units [10] and migrate to different areas of the cell [11]. PKA components have been found in the mitochondria of some species and tissues [2], for example, in the mouse oocyte [12].
PKA enzyme activity has been found in the mitochondria of human placenta and the enzyme appears to contribute to the phosphorylation of a 20 kDa mitochondrial protein (MP20). Modulation of protein phosphorylation in the mitochondria may regulate the physiological processes that mitochondria are involved in, in different tissues such as apoptosis, ATP generation and steroid hormone synthesis [2, 13]. The finding of PKA enzyme activity in placental mitochondria led to the speculation that PKA is secured in this location by an AKAP [2]. Furthermore, it has been suggested that this placental and mitochondrial PKA may phosphorylate and activate important modulators of placental physiology such as the Steroidogenic Acute Regulatory protein (StAR) [2, 14]. Such an activation of StAR may be able to increase StAR’s regulation of cholesterol transport from the outer mitochondrial membrane to the inner membrane where it is converted into progesterone in the first and rate-limiting step in steroidogenesis [15-17].
A vital developmental event for the placenta is the differentiation of trophoblast cells into cytotrophoblast and syncytiotrophoblast [18]. The placental steroid hormones that are vital for pregnancy to progress successfully to term are produced in the syncytiotrophoblast [19]. Mitochondria from the syncytiotrophoblast are lighter than those of the cytotrophoblast and therefore centrifugation can be used to separate the organelles from either cell type of cell [20, 21]. In this study we used specific antibodies to investigate the possibility that PKAa Cat and AKAP79 are present in various locations in the human placenta including mitochondria from both cytotrophoblast and syncytiotrophoblast.
MATERIALS & METHODS
Isolation of Mitochondria from Human Placenta
Human term placentae were collected from the Royal Prince Alfred Hospital, Sydney, and perfused in chilled phosphate saline buffer (PBS) to remove blood. Mitochondria were isolated as described by Corso and Thomson [21], briefly, the placentae were placed in chilled PBS and the tissues from the maternal side of the placenta were diced into pieces of approximately 1cm3. The diced tissues were homogenised in homogenisation buffer containing: 20mM Tris-HCl, 210mM mannitol, and 70mM sucrose. The homogenate was then centrifuged (Beckman J2-21M/E Centrifuge) at 700 × g for 10 minutes at 4ºC to remove cell debris and nuclei, for brevity the pellet from this stage will be referred to as nuclei. The supernatant was decanted and stored at 4ºC, and the pellet was resuspended twice using the homogenisation buffer and centrifuged at 700 × g for 10 minutes at 4ºC. The combined supernatants were centrifuged at 9750 × g for 15 minutes at 4ºC to yield the mitochondrial pellet. The mitochondria were washed free of microsomes and cytosol in the supernatant three times by re-suspension, using a dounce homogenizer, in homogenisation buffer and centrifuged at 9750 × g for 15 minutes at 4ºC. For brevity the supernatant at this stage will be referred to as microsomes. After three washes the supernatants were removed and the mitochondria pellet was re-suspended in the homogenisation buffer. This preparation is referred to as mitochondria.
Separation of Heavy and Light Mitochondria
Isolated mitochondria from human placentae were separated into heavy mitochondria characteristic of cytotrophoblast mitochondria and light mitochondria characteristic of syncytiotrophoblast mitochondria by sequential centrifugation following the method of Martinez and colleagues [20]. Briefly, mitochondria were resuspended in an equal volume of homogenisation buffer and centrifuged at 4000 × g for 15 minutes at 4ºC. The pellet was designated as heavy mitochondria. The pellet was removed and the supernatant was further centrifuged at 16,000 × g for 15 minutes at 4ºC to obtain light mitochondria.
Fractionation of Mitochondria
To produce vesicles of inner membrane, outer membrane and vesicles of outer and inner membrane joined by a contact site, whole unbroken mitochondria were processed by an established swelling and shrinking procedure and sucrose gradient separation technique [22-24]. Briefly, mitochondria in homogenisation buffer were centrifuged at 9750 × g for 15 minutes at 4ºC to remove the excess buffer then subjected to swelling and shrinking by resuspension and incubation in 7.5ml of 10mM phosphate buffer at 4ºC for 20 minutes, followed by the addition of 3ml of 60% sucrose and further incubated at 4ºC for 20 minutes. Mitochondrial membranes were further disrupted by sonication using Sonifier B-12 (Branson Sonic Power Company) three times for 30 seconds at level 6 with 30 second intervals in between. Unbroken mitochondria were removed by centrifugation (using Beckman J2-21M/E Centrifuge) at 9750 × g for 20 minutes at 4ºC. This process produces vesicles of inner membrane that are generally relatively large, smaller vesicles of outer membrane and vesicles of outer membrane joined at a contact site to a vesicle of inner membrane and these complexes are mainly intermediate in size. 5ml aliquots of suspensions of vesicles (with protein concentration of approximately 5mg/ml) were layered on a 23ml, 24.4%-61.2% continuous sucrose density gradient. Sucrose gradients were subjected to ultracentrifugation (using Beckman XL-90 Ultracentrifuge) at 100,000 × g for 20 hours at 4ºC. The gradient was then collected as 20 fractions of 1.4ml each, starting at the bottom of the tube. The majority of inner membrane is found at the bottom of the tube, the outer membrane is located mainly towards the top of the tube and the contact sites are located between the inner and outer membrane [22, 23, 16, 24]. The positions of outer and inner membrane were checked to be the same as previous implementations of this procedure using enzyme markers [24].
Protein Concentration Estimation
The protein concentration of samples from each placenta was assayed using the Pierce Bicinchoninic acid (BCA) Protein Assay Kit and Bio-Rad Microplate Manager (Version 5.0.1) as per manufacturer instructions.
Sodium Dodecyl Sulphate Polyacrylamide Gel Electrophoresis (SDS PAGE)
All samples were diluted so as to contain an equal amount of protein. Samples containing a total of 20 ìg of protein were loaded onto a 12% SDS gel and run at a voltage of 100V using the Biorad Protean III System according to the manufacturer until the bromophenol blue dye font reached the bottom of the gel (approximately 2 hours). For sucrose gradient samples the first 19 fractions were loaded leaving one lane free for standard molecular weight markers. Protein bands were detected using Coomassie blue stain. Where a representative single lane from several repeats is shown in a diagram, the lane shown is that of the first sample analysed. There was no post result selection of lanes for diagrams.
Western Blotting
Proteins were transferred to nitrocellulose membrane (100V for 1 hour) using a Mini Trans-Blot system (Bio-Rad laboratories) according to manufacturer’s instructions. Complete transference of colour coded molecular weight standards from the gel to the nitrocellulose membrane was checked. The nitrocellulose membrane was blocked with 5% skim milk in 1% phosphate-buffered saline (1% PBS) for 1 hour. Following the blocking of non-specific binding the nitrocellulose membrane was incubated with primary antibody for 1 hour. The primary antibodies used were PKAα Cat (C-20) rabbit polyclonal IgG antibody (Santa Cruz) and AKAP79 (H-105) rabbit polyclonal IgG antibody (Santa Cruz). The membranes were given 2 quick rinses in phosphate-buffered saline containing 0.05% (v/v) Tween-20 (TPBS), 4 × 5 minutes washes with TPBS, and 30 minutes incubation in secondary antibody. The membrane was then given 2 quick rinses in TPBS, 4 × 5 minutes washes in TPBS, 1 rinse in PBS and then incubated in chemiluminescent substrate (Pierce SuperSignal West Pico) as per the manufacturer’s instructions. The membranes were then exposed to film (Pierce Cl-Xposure) for various times and were analysed using the public domain computer program ImageJ, a Java conversion of NIH Image developed at the U.S. National Institutes of Health and available on the Internet at http://rsb.info.nih.gov/nih-image/).
Statistical Analysis
Results were analysed by paired t-test assuming equal variance and a P value of 0.05 or less was considered significant.
RESULTS
Isolation of Cellular Fractions from Human Placenta
Proteins in the, crude homogenate, nuclei, microsomes and mitochondria from three different human placentae were analysed using SDS PAGE and detected using Coomassie blue (Fig. 1). The homogenate, nuclei, microsomes and mitochondria showed protein band patterns, which spanned the 7-215 kDa molecular weight range. Densitometry analysis of the bands displayed in Fig. (1A) showed that the total placenta homogenate and microsomes displayed major peaks at 65 and 10 kDa that were not present in mitochondrial fractions (Fig. 1B).
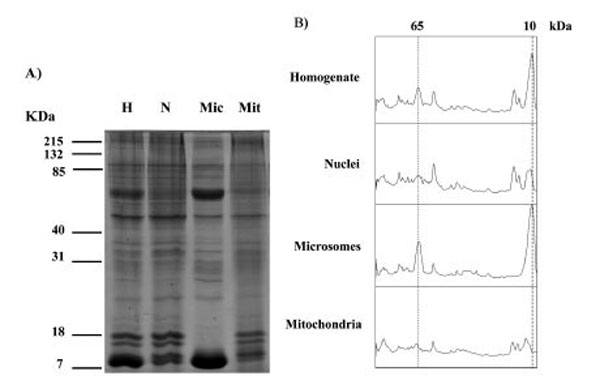
SDS PAGE analysis of proteins in homogenate (H), nuclei (N), microsomes (Mic) and mitochondria (Mit) fractions from human placenta. Similar results were observed in a total of 3 different placentae. Gels stained with Coomassie are shown in A), densitometry scans of the lanes are shown in B) the positions at 65 kDa and 10 kDa are indicated with dotted lines.
PKAα Cat expression in Human Placental Tissue
When Western blots of nuclei, microsomes and mitochondria were probed with the PKAα Cat antibody they all displayed a major band of immunoreactivity at approximately 44 kDa (Fig. 2). A minor band was observed at an approximately 90 kDa. One sample of each cellular fraction from three different placentae was analysed, and similar results were observed in three different human placentae (Fig. 2). The intensity of the 44 kDa PKAα Cat band in microsome fractions was significantly higher than in mitochondria (p = 0.02). On the other hand, there was no significant difference in the band intensity between nuclei and mitochondria (p = 0.30), and nuclei and microsomes (p = 0.23).
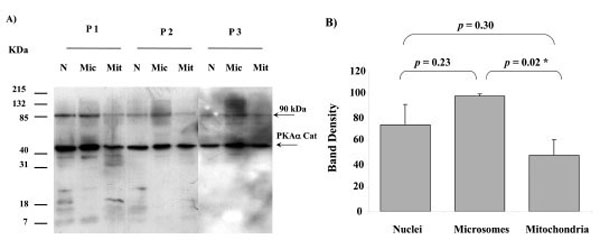
PKAα Cat expression in nuclei (N), microsomes (Mic) and mitochondria (Mit) from 3 different human placentae, (P1, P2 and P3). For each placenta one sample from each fraction was analysed. SDS PAGE, Western blotting and autoradiography results shown in A). A major protein band with approximate size of 44 kDa was detected in all fractions by the PKAα Cat antibody. A minor band at approximately 90 kDa was also present. The relative intensity taken as percentage of the most intense band for each placenta (values represent the mean ± S.E.) of the 44 kDa bands shown in A) are plotted in B). An asterisk indicates a significant difference with a p value of less than 0.05.
PKAα Cat expression in Heavy And light Mitochondria
To determine the difference in the level of PKAα Cat expression in heavy and light placental mitochondria, isolated mitochondria were separated into heavy and light mitochondria as described in methods and materials. Each placenta (of a total of 5) yielded one isolate of heavy mitochondria and one isolate of light mitochondria. From each isolate (from each placenta) four aliquots were taken for SDS PAGE analysis and Western blotting. The process was repeated a total of five times, each of these times using a different placenta.
The intensities of the PKAα Cat immuno-reactive bands at 44 kDa in heavy and light mitochondria were compared for isolates from each placenta, (Fig. 3). There was no significant difference in the band intensity for the 44 kDa PKAα Cat immuno-reactive band between the heavy and light mitochondria in four out of five human placentae (p = 0.94 for placenta 1, p = 0.96 for placenta 2, p = 0.16 for placenta 3, p = 0.40 for placenta 5). There was a significant difference in the band intensity for the 44 kDa PKAα Cat immuno-reactive band between the heavy and light mitochondria in human placenta 4 (p = 0.02).
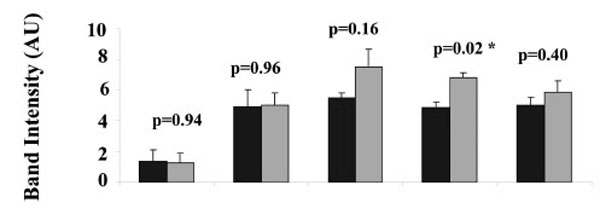
Comparison of PKAα Cat expression in heavy (H) and light (L) mitochondria. For each of 5 placentae one preparation of heavy and one preparation of light mitochondria was produced. For each preparation of light or heavy mitochondria, three aliquots were taken for SDS PAGE and Western blot, the intensities of the three 44 kDa protein bands in each preparation of heavy and light mitochondria from five different human placentae are shown. Values represent the mean ± S.E. of triplicate samples ■ - heavy mitochondria; ■- light mitochondria. An asterisk indicates a significant difference with a p value of less than 0.05.
AKAP79 Expression in Human Placental Tissue
Western blots of nuclei, microsomes and mitochondria all displayed a major band of AKAP79 immunoreactivity at the approximately 80 kDa region using AKAP79 antibody (Fig. 4). The intensity of this protein band detected in nuclei, microsomes and mitochondria from three different human placentae are shown in Fig. (4B). There was no significant difference in the band intensity between nuclei, microsomes and mitochondria (nuclei and microsomes: p = 0.18; nuclei and mitochondria: p = 0.89; microsomes and mitochondria: p = 0.09) (Fig. 4B).
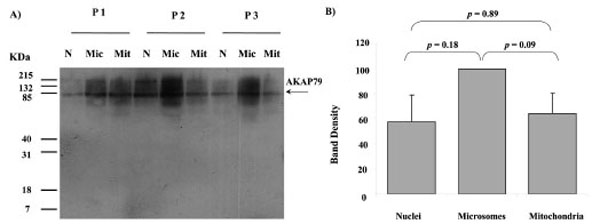
AKAP79 immunoreactivity in nuclei (N), microsomes (Mic) and mitochondria (Mit) from 3 different human placentae, (HP 1, 2 and 3). SDS PAGE, Western blotting and autoradiography results shown in a). A protein band of approximate size 80 kDa was detected in all fractions by the AKAP79 antibody (A). The relative intensity taken as percentage of the most intense band for each placenta (values represent the mean ± S.E.) of the 80 kDa bands shown in A) are plotted in B)
AKAP79 Expression in Heavy and Light Placental Mitochondria
To determine the difference in the level of AKAP79 expression in heavy and light placental mitochondria, isolated mitochondria were separated into heavy and light mitochondria as described in methods and materials. Each placenta (of a total of 5) yielded one isolate of heavy mitochondria and one isolate of light mitochondria. From each isolate (from each placenta) four aliquots were taken for SDS PAGE analysis and Western blotting. The process was repeated a total of five times, each of these times using a different placenta. The AKAP79 immuno-reactive band was present in both heavy and light mitochondria in all placentae (Fig. 5). The band intensity of the AKAP79 band was not significantly different as compared between the heavy and light placental mitochondria in any of the human placenta (p = 0.81 for placenta 1, p = 0.79 for placenta 2, p = 0.21 for placenta 3, p = 0.73 for placenta 4, p = 0.37 for placenta 5).
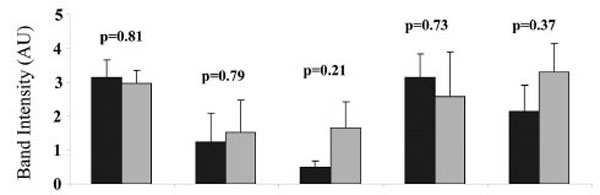
Comparison of the AKAP79 expression in the heavy (H) and light (L) mitochondria. For each of 5 placentae one preparation of heavy and one preparation of light mitochondria was produced. For each preparation of light or heavy mitochondria, three aliquots were taken for SDS PAGE and Western blot. The intensities of the AKAP79 immuno-reactive protein bands in each preparation of heavy and light mitochondria from five different human placentae are shown. Values represent the mean ± S.E. of triplicate samples ■ - heavy mitochondria; ■- light mitochondria.
PKAα Cat Immunoreactivity in Human Placental Submitochondrial Fractions
When submitochondrial fractions were separated on sucrose gradients and analysed using SDS-PAGE and Western blot, PKAα Cat immuno-rearctivity peaked at fraction 17 in the outer membrane region of the gradient (Fig. 6).
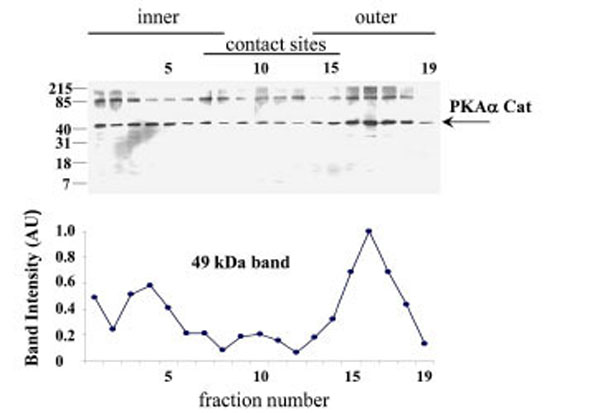
PKAα Cat expression in the human placental mitochondrial fractions. PKAα Cat expression was detected using SDS-PAGE, Western blotting and autoradiography (Exposure time = 1 minute). The PKAα Cat immuno-reactive bands are shown in A), the intensities of the 44 kDa PKAα Cat bands are shown in B). The experi-ment was repeated for a total of three different placentae with similar results.
AKAP79 Cat Expression in Human Placental Submitochondrial Fractions
When submitochondrial fractions were separated on sucrose gradients and analysed using SDS-PAGE and Western blot, AKAP79 immuno-reactivity peaked at fraction 12 in the contact site region of the gradient (Fig. 7).
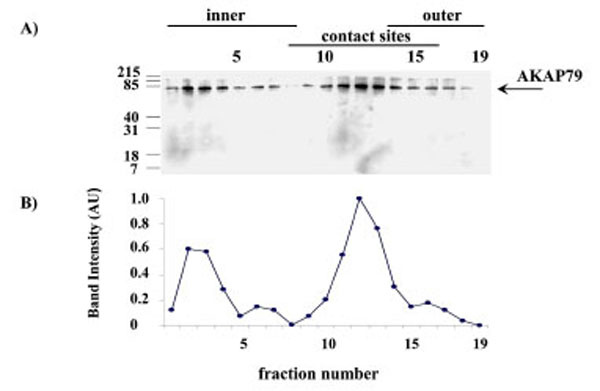
AKAP79 immunoreactivity in the human placental mitochondria fractions. AKAP79 immunoreactivity was detected using SDS-PAGE, Western blotting and autoradiography (Exposure time = 1 minute). The AKAP79 immuno-reactive bands are shown in A) the intensity of the AKAP79 bands are shown in B). The experiment was repeated for a total of three different placentae with similar results.
DISCUSSION
The method used in this study to fractionate cellular components has been shown to produce mitochondria free of any significant contamination from microsomes using the ras protein as an indicator, ras is present in the microsome fraction but is undetectable in the mitochondria fraction [25]. In the present study we again checked the purity of the mitochondrial fraction using the 65 and 10 kDa proteins that are present in the microsome and homogenate fractions but absent from the mitochondria fraction (Fig. 1).
Prior to this study PKA enzyme activity has been shown to be present in human placental mitochondria [21] but whether this bioactivity was exerted by a version of PKA that contained an α catalytic subunit was not known. Indeed there has been no prior study that has shown what PKA subunits are present in any cellular location in human placenta. In this study the presence of PKAa Cat positive bands of immunoreactivity in all three cellular subfractions studied (Fig. 2A) indicates that PKAa Cat is ubiquitous in the human placental cells and that levels of PKAa Cat in the mitochondria are of similar concentrations to that found in the nuclei (Fig. 2B).
The molecular weight estimation by SDS PAGE of approximately 44 kDa for PKAa Cat is the same reported by the antibody manufacturer when detecting PKAa Cat in mouse brain extract (http://datasheets.scbt.com/sc-903.pdf) and similar to the estimated molecular weight of PKAa Cat previously found in the cytoplasm of human myometrium (approximately 42 kDa) [8]. SDS PAGE has an accuracy in molecular weight determination of up to approximately +/- 10% [26]. Interestingly, an additional minor PKAa Cat band at 90 kDa was detected by the PKAα Cat antibody in mitochondria as well as nuclei and microsomes (Fig. 2). The minor 90 kDa band may indicate some aggregation of PKAa Cat or the presence of a larger weight precursor protein.
Cytotrophoblast mitochondria are heavier than syncytiotrophoblast mitochondria [20] and the finding that the level of PKAα Cat immunoreactivity was not significantly different in light mitochondria as compared to heavy mitochondria in four out of five placentae tested (Fig. 3) suggests that syncytiotrophoblast mitochondria do not need significantly more PKA than cytotrophoblast mitochondria for physiological uses of PKA in the organelle. Previous studies using phosphotyrosine antibodies have demonstrated that while some phosphotyrosine proteins are evenly distributed between heavy and light mitochondria, the concentrations of two phosphoproteins of 20 and 16 kDa molecular weight was significantly higher in heavy mitochondria. Additionally, the amount of porin, the protein that makes up the voltage dependent anion channel in mitochondria is significantly higher in heavy mitochondria (Ma and Thomson unpublished results). It will be interesting to determine in future studies what physiological roles PKA is playing in the mitochondria of both syncytiotrophoblast and cytotrophoblast.
For PKA to be involved in physiological processes in the mitochondria of human placenta it would be expected that an AKAP would be present in the organelle to fasten the protein in the organelle [2]. Figs (4, 5) and (7) indicate that human placental mitochondria contain at least one AKAP, AKAP79 at concentrations that are similar to those found in the nuclei and microsome fractions (Fig. 4). This AKAP79 displays a similar migration distance in SDS PAGE compared to AKAP79 in other cells such as neuroblastoma and myoblast cell lines [27]. Both cytotrophoblast and syncytiotrophoblast mitochondria appear to contain this AKAP at similar concentrations and future studies may reveal the role played by AKAP79 in both types of mitochondria. Fig. (5) indicates that both heavy and light mitochondria contain similar amounts of AKAP79 immunoreactivity and it will be important in future investigations to determine whether AKAP79 is binding PKA subunits with active enzyme activity in both heavy and light mitochondria of human placenta.
The pattern of PKAα Cat immunoreactivity in sucrose gradient separations of submitochondrial fractions suggests that PKAα Cat is located predominantly in the outer membrane (Fig. 6). AKAP79 on the other hand appears to be located predominantly in contact site vesicles (Fig. 7) and so it appears that the majority of AKAP79 is not responsible for anchoring the majority of PKAα Cat in these mitochondria. It is possible that another species of AKAP may be anchoring substantial quantities of the PKA in placental mitochondria. Submitochondrial fractionation on sucrose gradients are useful to show where the majority of a protein is in the mitochondrion but because of the heterogeneity in size of submitochondrial particles the technique cannot fully purify inner membrane from outer membrane and cannot quantify the relative amounts of a protein in different mitochondrial locations (see Thomson for review) [16]. Therefore the possibility remains that smaller amounts of AKAP79 in the outer membrane may contribute to the anchoring of PKA in this region.
Indeed, there is a paucity of information on the protein kinase and AKAP species that are present in the human placenta and it will be important in future studies to determine what combinations and configurations of kinase subunits and anchors are present in the cells and sub-cellular locations of the organ. Future experiments using electron microscopy and immuno-gold labelling [28] may help to show what AKAP species are co-localised with PKA in the mitochondria. In some steroidogenic tissues phosphorylation of the steroidogenic acute regulatory protein (StAR) in or close to the mitochondria by PKA is thought to accelerate cholesterol transport to the inner membrane for conversion to pregnenolone [29-32] and AKAP121 appears to facilitate steroidogenesis in the mouse leydig cell line MA-10 [33].
CONCLUSION
This study has indicated that PKAα Cat and AKAP79 are present in the mitochondria of both cytotrophoblast and syncytiotrophoblast. PKAα Cat it is located mainly in the outer membrane whereas AKAP79 is located predominantly in the contact sites. Future experiments are necessary to establish the physiological roles of PKAα Cat and AKAP79 in the mitochondria of human placenta.
CONFLICT OF INTEREST
None declared.
ACKNOWLEDGEMENTS
We would like to thank the staff of the Women and Babies Service at The Royal Prince Alfred Hospital for help in collecting the placentae. This study was conducted under the Royal Prince Alfred Hospital Human Ethics Committee protocol X06-0061and funded by a grant from the Integrative Physiology Group, The University of Sydney.
ABBREVIATIONS
REFERENCES
[PubMed Link]
[PubMed Link]
[PubMed Link]
[PubMed Link]
[PubMed Link]
[PubMed Link]
[PubMed Link]
[PubMed Link]
[PubMed Link]
[PubMed Link] [PMC Link]
[PubMed Link]
[PubMed Link]
[PubMed Link]
[PubMed Link]
[PubMed Link]
[PubMed Link]
[PubMed Link]
[PubMed Link]
[PubMed Link]
[PubMed Link]
[PubMed Link]
[PubMed Link]
[PubMed Link]
[PubMed Link]