All published articles of this journal are available on ScienceDirect.
Sphingosine Increases ATP Release From Red Blood Cells
Abstract
Background:
RBC plays a pivotal role in oxygen delivery, improving distribution where it needs. When RBC enters a low oxygen area, a mechanism mediated by a signaling pathway releases ATP, responsible for vasodilatation.
Objective:
Clarify the potential role of sphingosine on the release of ATP from RBC.
Methods:
ATP release increases after sphingosine exposure in RBC under low oxygen conditions. ATP release in deoxygenated RBC shows data like that of control RBC: (1) RBC after band 3 modification by 4,4'- diisothio-cyanato-stilbene- 2,2'-disulphonic acid (DIDS); (2) CO-treated RBC.
Unlike phosphofructokinase, adenylate cyclase (AC) activity increases after exposure to sphingosine.
Results:
We show that cAMP synthesis and ATP release are not failed in sphingosine-treated red blood cells in response to incubation with mastoparan 7, forskolin plus 3-isobutyl-1-methyl xanthine, agents that stimulate cAMP synthesis.
Conclusion:
Deoxy-hemoglobin, band 3, and AC are involved in the signaling pathway responsible for ATP released after sphingosine exposure.
1. INTRODUCTION
Adenosine triphosphate (ATP) plays a pivotal role in several pathways [1]. ATP activates P2 receptors on endothelium, platelets [2, 3], and other blood cells [4], regulating several effects [5]. Recent papers support the hypothesis that red blood cells (RBC) are involved in the mechanism responsible for vasodilatation [5-7]. RBC can release ATP in low oxygen areas following deformation [8-13], thus modulating vasodilation [14-17].
ATP released from RBC, can stimulate NO synthesis [9, 14, 15], responsible for vasodilation [18], as also reported in isolated rat cerebral arterioles [16]. ATP released from RBC involves several proteins, including G proteins [19-21], adenylyl cyclase (AC), cyclic AMP-dependent protein kinase A [13], cystic fibrosis transmembrane conductance regulator, and protein pannexin [22, 23]. In RBC from type 2 diabetes and cystic fibrosis subjects, alterations of these proteins were reported [24, 25]. In RBC, Gi stimulation increases AC activity, leading to increased cAMP synthesis and ATP release [19-21]. ATP release causes vasodilation by NO and activation of P2Y receptors on endothelial cells [26]. ATP released from the low oxygen area involves protein band 3 [27]. Sphingosine 1 phosphate (S1P), generated by Sphk1 [28], promotes deoxy-Hb (deoxy-hemoglobin) binding to band 3, increasing glycolysis flux [29]. Previous studies demonstrated that extracellular S1P is involved in many physiological activities [30]. Here, we investigate the hypothesis that exposure of RBC to sphingosine alters the signal transduction pathway relating to ATP released from RBC in response to reduced oxygen tension. ATP release into the vasculature cause vasodilation and accelerated transit of the hypoxic RBC to the lungs.
2. MATERIALS AND METHODS
2.1. Chemicals and Preparation of RBC
Sphingosine and other chemicals have been obtained from Sigma Aldrich. According to the Declaration of Helsinki, heparinized fresh human blood has been obtained from informed, healthy donors. Plasma separation was obtained by centrifuging at 2500g for 5 minutes. Human RBCs have been isolated by density gradient centrifugation by Ficoll. Samples have been prepared as previously reported [31]. Two minutes after deoxygenation, a 200-µl sample of the buffer-containing cells has removed for cAMP and ATP analysis. Saturation of hemoglobin was measured as reported by Zijlstra [32]. In low oxygen conditions, deoxy-Hb was 60% ± 0.32%. Sphingosine concentration has been fixed to 200 nM. To exclude the possibility that RBC lysis contributes to the measurement of ATP release, the presence of hemoglobin in the supernatant was determined by light absorption at 405 nm (Cary 3E, Varian, Palo Alto, CA)
2.2. Measurement of ATP
ATP was measured by the luciferin-luciferase technique as previously reported [8, 9], which uses the ATP concentration dependence of light generated by the reaction of ATP with firefly tail extract.
2.3. Measurement of cAMP
cAMP concentration has been determined by a cAMP Biotrak enzyme immunoassay system (Amersham Biosciences).
2.4. Incubation of RBC with Agents that Alter cAMP Concentration or Activity
Treated and untreated RBC has incubated for 2 minutes with either mastoparan 7 (activator of Gi, 10 µmol/l) [13] or forskolin (10 µM, dissolved in ethanol, final ethanol concentration 0.2%) to stimulate adenylyl cyclase, plus 3-isobutyl-1-methyl xanthine (IBMX) (100 µM), and prevent cAMP degradation or their respective vehicles. cAMP and ATP levels measured have normalized to an RBC count of 2 × 105 cells/mm3.
2.5. Phosphofructokinase Activity
Phosphofructokinase (PFK) enzymatic activity has been determined by the method described previously [33].
2.6. Experiments with Band 3 Inhibitor
Experiments with band 3 inhibitor, i.e., 4,4'- diisothio-cyanatostilbene- 2,2'-disulphonic acid (DIDS), were performed according to the method of Jennings and Passow [34]. A freshly prepared 12μM DIDS solution has been added to the RBC suspensions to reach a final DIDS concentration of 0.5 μM. Hemoglobin spectra were acquired by Zijlstra et al. [32].
2.7. Experiments with Carbon Monoxide-Saturated RBC
Deoxygenated cells were flushed twice with an absolute carbon monoxide atmosphere (760 Torr) and then brought under a nitrogen atmosphere. The vials were then incubated at 37°C, and one control vial was used to determine the carboxy-Hb content, as reported by Zijlstra et al. [32]. The controls for pH values and hemolysis, done as previously described, provided the same results as those obtained in the case of LOS and HOS samples.
2.8. Statistical Analysis
The data were analyzed by one-way ANOVA, followed by a post-hoc Newman-Keul test for multiple comparisons among group means, and differences were considered statistically significant if P < 0.05. Unless otherwise specified, all results are presented as the mean ±SD of at least three different experiments performed in triplicate.
3. RESULTS
3.1. Effect of Sphingosine on ATP Release
In Fig. (1), ATP released values are significantly higher in cells exposed for 24 hours to solutions with low oxygen saturation than when treated in control conditions. To clarify the role of band 3 in the sphingosine-mediated effects, experiments with an inhibitor of band 3, i.e., 4,4'- diisothio-cyanatostilbene- 2,2'-disulphonic acid (DIDS) function, were performed. Under these conditions, RBC in low oxygen conditions showed a pronounced reduction of ATP release, whereas RBC treated in high oxygen conditions was only slightly altered (Fig. 1). In RBC, ATP release links to a pathway involving Gi and adenylyl cyclase (AC) [19-21]. Mastoparan 7 and forskolin, plus IBMX, both Gi and cAMP synthesis activators, respectively, have been used to clarify the mechanism responsible for sphingosine-mediated effects on ATP release from RBC under low oxygen conditions. As shown in Fig. (2), in the presence of sphingosine, ATP release values were not different between RBC in mastoparan 7 or with forskolin, plus IBMX to control cells. ATP release was determined in RBC saturated with carbon monoxide (CO saturation greater than 90%), i.e., Hb in the fully liganded R conformation and incubated in low oxygen conditions, to clarify the role of Hb involvement in the effect of sphingosine. As shown in Fig. (1), ATP release values were like those measured in RBC in high oxygen conditions, either in the presence or absence of sphingosine.
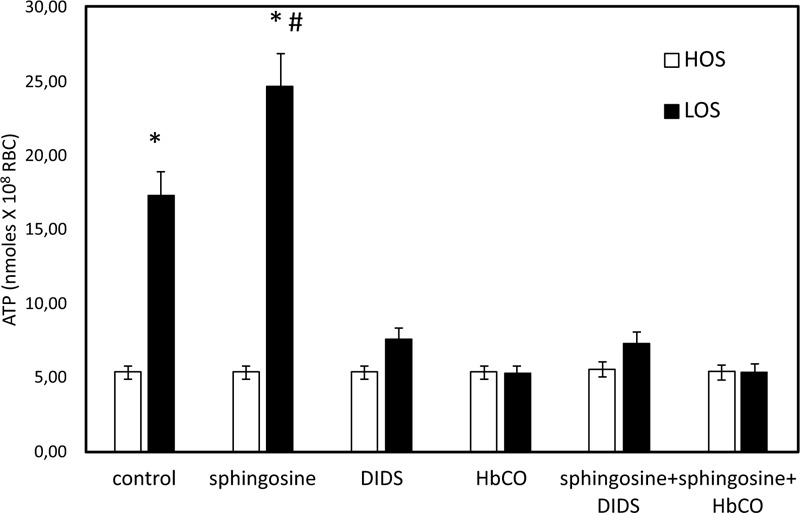
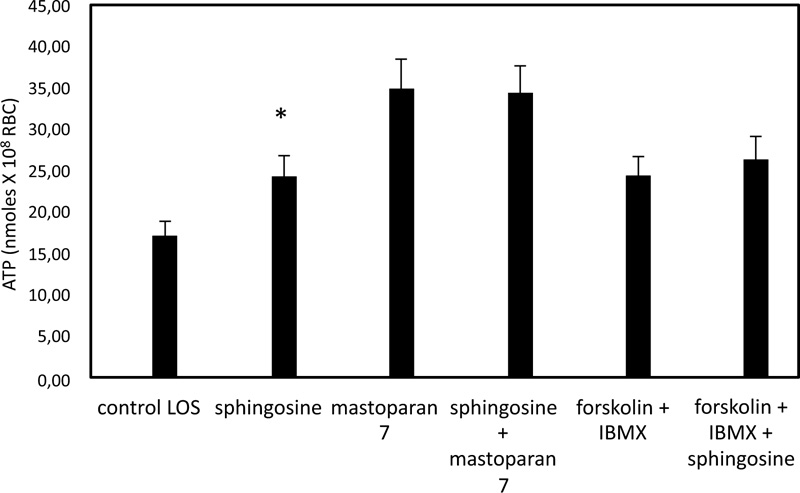
3.2. Effect of Sphingosine on the Accumulation of cAMP
In pathological RBC, low basal cAMP levels correlate with a reduced ability to release ATP from RBC under hypoxic conditions [35]. cAMP levels in sphingosine-treated RBC are significantly higher when the cells are exposed to solutions with low oxygen than in control conditions (Fig. 3). In RBC, it has been shown that activation of Gi results in stimulation of adenylate cyclase (AC) activity, leading to increased cAMP synthesis and release of ATP [19-21]. Further experiments were performed with mastoparan 7 and forskolin, plus IBMX, agents able to stimulate cAMP synthesis by activating Gi and AC, respectively. As shown in Fig. (4), cAMP levels are not different between RBC incubated with mastoparan 7 or with forskolin, plus IBMX in the presence and absence of sphingosine.
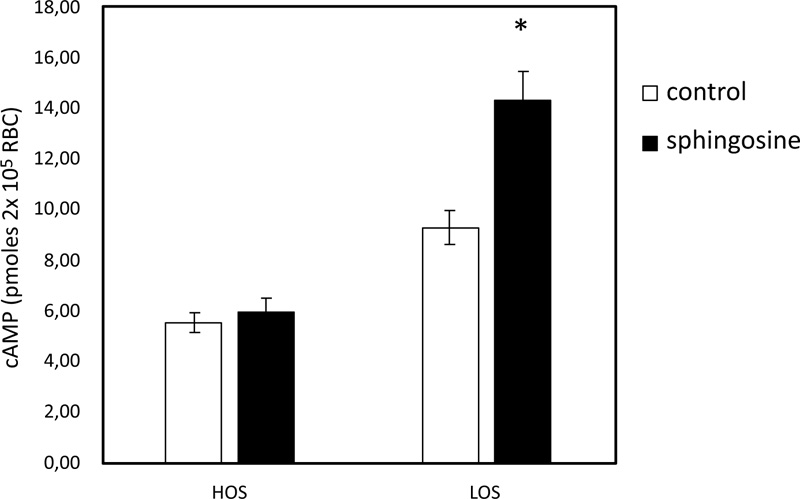
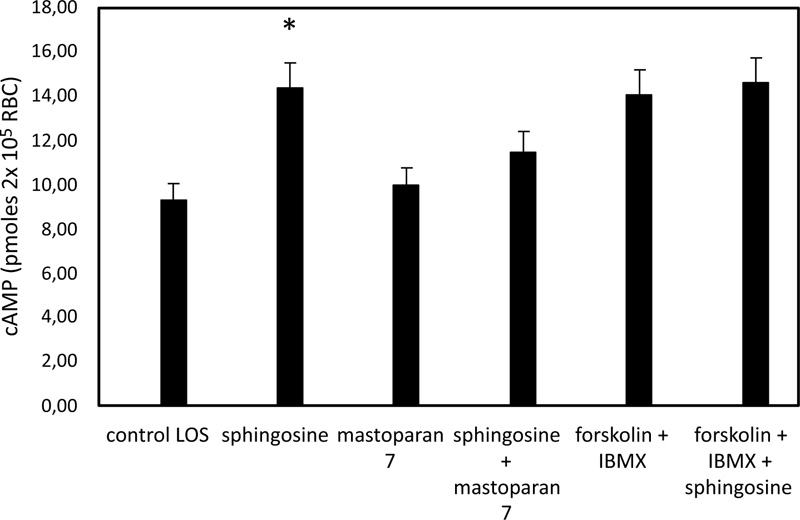
3.3. Effect of Sphingosine on PFK Activity
To test the possibility that ATP release following sphingosine exposure might be linked to an alteration of phosphofructokinase (PFK) activity, we performed an analysis of the activity of this enzyme on hemolysates obtained from treated and controlled intact RBC. PFK activity based on the three parameters Vmax, S50, and n50, did not show significant differences between controls and sphingosine-treated cells. In Vmax (89.53 ±4.18 and 83.57 ± 3.24 mM. min/gHb for control and treated cells, respectively), n50 (1.29± 0.29 and 1.29 ± 0.19 mM for control and treated cells, respectively) and S50 (0.240 ± 0.126 and 0.340± 0.123 for control and treated cells, respectively).
4. DISCUSSION
Released ATP from RBC following reduced oxygen and mechanical deformation plays a key role in regulating vascular resistance in the lung, striated muscle, and isolated cerebral arterioles [9, 14, 15, 35, 36]. Interaction between deoxyhemoglobin and protein band 3 triggers the mechanism responsible for ATP released from RBC [27, 37]. In line with this finding, alteration of the cytoskeleton and signaling pathways are related to the oxygenation/ status of hemoglobin [38-41]. Our data show that following sphingosine exposure under low oxygen conditions, RBC increases cytosolic cAMP levels and ATP released from RBC. Here, we show that the increase of ATP released from RBC incubated at low oxygen tension in the presence of sphingosine was strongly reduced when RBC was incubated with an inhibitor of band 3 activity (DIDS) and under experimental conditions with HbCO, suggesting the involvement respectively of protein band 3 and Hb-deoxy in the sphingosine-mediated effects observed in RBC incubated low oxygen tension. The absence of any significant effect of sphingosine on phosphofructokinase (PFK) activity rules out the glycolytic flux's involvement in the observed sphingosine-mediated effects. When heterotrimeric G proteins are activated, the subunit dissociates from the ß- complex. The subunit and the ß- complex can then regulate, either individually or synergistically, the catalytic activity of adenylate cyclase (AC) [19-21, 42]. Here, we evidence that in sphingosine-treated RBC, cAMP levels are higher for control cells, suggesting that the increased ATP release in sphingosine-treated RBC under low oxygen conditions could be explained by increased activity of AC. It should also be noted that mechanical deformation stimulates ATP released from RBC [43]. Thus, it is reasonable to hypothesize that sphingosine may affect this mechanism of ATP release. Further studies are required to confirm such speculation.
CONCLUSION
It has been shown that sphingosine exposure to RBC triggers an increase in ATP released from deoxygenated RBC by a mechanism started by deoxy Hb/band 3 binding, as suggested by experiments performed with DIDS and HbCO. This finding implies that ATP released from RBC induced by sphingosine may be a novel mechanism for adaptation to hypoxia and confirms a key role for intracellular sphingosine in RBC physiology. It opens new opportunities to counteract vascular hypoxia, occurring in several diseases.
ETHICS APPROVAL AND CONSENT TO PARTICIPATE
Not applicable.
HUMAN AND ANIMAL RIGHTS
No animals/humans were used for studies that are the basis of this research.
CONSENT FOR PUBLICATION
Not applicable.
AVAILABILITY OF DATA AND MATERIALS
Not applicable.
FUNDING
This research was supported by grants from the University of Cassino (FAR 2019).
CONFLICT OF INTEREST
The author declares no conflict of interest, financial or otherwise.
ACKNOWLEDGEMENTS
Declared none.