All published articles of this journal are available on ScienceDirect.
β-Glucan Improves Conditions of Chronic Fatigue in Mice by Stimulation of Immunity
Abstract
Background:
Various natural molecules have been studied for the enhancement of physical endurance. Glucan has been found to improve various stress-related conditions and to improve fatigue and endurance.
Objective:
In this study, we evaluation of glucan effects on some reactions involved in chronic fatigue has been focused.
Methods:
Phagocytosis of neutrophils, the production of IL-2, IL-4, and IL-10 by spleen cells, and levels of antioxidant glutathione and oxidative stress marker superoxide dismutase in brain were measured. In addition, the effects of glucan on water immersion and on rotarod were also measured.
Results:
The glucan supplementation strongly improved the suppressed phagocytosis and changes in cytokine and levels of oxidative stress markers caused by fatigue. In addition, glucan supplementation also increased the motor functioning of tested animals.
Conclusion:
Our data suggested that anti-fatigue properties of glucan are related to its well-established effects as stimulator of immune reactions.
1. INTRODUCTION
β-Glucans are polymers of glucose extracted from yeast cell walls, bacteria, fungi and cereals such as oats and barley [1]. β-Glucans from yeast and fungi consist of a backbone of glycopyranosyl molecules joined by 1,3-β-links; from this backbone, side chains can be joined by 1,6-β-links, producing a branched molecular structure. Fungal β-glucans have short branches while they are long in yeast. Cereal cell wall consists of not branched β-glucans with glucopyranose molecules linked by 1,3-β and 1,4-β linkages. On the other hand, bacterial β-glucans are unbranched with only 1,3-β-linkages between the glycopyranosyl molecules; similarly, barley and most cereal grains contain unbranched ß-glucan chains [2]. Until recently, biologically efficient β-glucans were supposed to have similar structures—a main chain of β-(1→3) bound D-glucopyranose molecules (for better perspective, imagine beads on the string) to which some D-glucopyranoses are randomly connected by β-(1→6) linkages causing a different degree of branching in different glucans. The detailed structure of β-glucans from dissimilar sources differs as well as their biological activity. In native β-glucans, their fibrils are composed from organized parts in which the main chain is coiled to a triple helix. These regions are combined with single or double filaments of β-(1→3)-D-glucopyranoses. The triple helix, formed by three hydrogen bonds and stabilized by side chains, is probably present only in high-molecular β-glucans with molecular weight over 90 kDa [3]. Diverse data on comparison of structure, molecular size, and biological effects can be found in literature.
β-Glucans are groups of dietary fibers and form the major structure found in the cell wall of yeast, fungi, algae, and cereal grains [4]. β-Glucans have been shown to stimulate the proliferation of white blood cells, which in turn stimulates the immune system. The β-glucans are transported to the small intestine, then passed through the Peyer’s patches in the GALT, and subsequently moved around the body. The presence of β-glucan is followed by its binding to specific receptors such as CR3 and Dectin-1 and results in stimulation of macrophages, higher formation of antibodies and increasing the activity of natural killer cells. In addition to direct stimulation of both specific and non-specific immunity, glucan can also influence expression of immune-related genes and proteins. The stimulation in immune functioning serves to combat the negative effects of enteric infection or immune suppression due to high stress rearing conditions. Health benefits of β-glucans have been exclusively documented over the past three decades with established effects on anti-inflammatory, anti-infectious and anti-cancer immunity (for review [5]). These effects are mediated via changes in numerous immunological mechanisms [6] including production of TNF-α [7, 8]. The role of TNF-α might be particularly important, as shown by a different model of fatigue [9]. These data resulted in recommendation of adding glucan to food [10, 11]. In addition, use of glucan as nanoparticles for vaccines is extensively studied [12].
Besides effects on the immune system and other direct biological effects, glucans were found to improve the negative effects of stress by reducing the level of corticosterone [13, 14], and to have positive effects following exercise stress [15]. In addition, β-glucan supplementations were found to play positive role in modulating exercise-induced changes in intensively training athletes [16], improve the cellular immune response, respiratory tract infections and mood in athletes [17, 18] and generally improve the exercise-induced problems of immune functions after heavy physical stress [19].
Various natural molecules have been studied for the enhancement of physical endurance. Gintonin, an active ingredient of ginseng, was recently shown to enhance performance of mice in rotarod test [20]. In mouse model, glucan attenuated chronic fatigue syndrome [21], whereas in human clinical trials, glucan supplementation resulted in improved endurance of children with chronic respiratory problems [22]. Glucan also has positive effects in cancer-related fatigue [23], probably via its anti-inflammatory action.
As prolonged and exhausting physical activity can cause immunosuppression [24], therefore, it was decided to evaluate the effects of β-glucan supplementation of various physiological and immunological parameters after severe physical stress.
2. MATERIAL AND METHODS
2.1. Animals
Female, 8 week old BALB/c mice were purchased from the Jackson Laboratory (Bar Harbor, ME). All animal work was done according to the University of Louisville IACUC protocol. Animals were sacrificed by CO2 asphyxiation. Nine mice/group were used.
2.2. Material
Yeast-derived insoluble Glucan #300 was purchased from Transfer Point (Columbia, SC). The purity is over 85%.
2.3. Experimental Groups
A control group of nine mice was administered PBS. The glucan-supplemented groups received different daily doses of glucan (50, 100 and 200 μg/mouse) orally from day 0 for 15 days after the start of swimming.
2.4. Cytokines
After being cultured in a humidified incubator (37o C, 5% CO2) for 72 hrs, levels of cytokines IL-2, IL-4 and IL-10 secreted by splenocytes in the presence of 5 μg Con A were determined by ELISA: Supernatants were collected, filtered through 0.45 μm filters, and IL-2 and IL-4 measured using Quantikine mouse kits (R&D Systems, Minneapolis, MN).
2.5. Biochemical Parameters
On the various points of the study, animals were sacrificed. The brains were removed, rinsed in isotonic saline solution and weighed. A 10% w/v tissue homogenates were prepared in 0.1 M phosphate buffer pH 7.4. The homogenates were centrifuged at 12 000 g for 60 minutes at 4oC. Glutathione (GSH) levels were estimated as described earlier [25]. Briefly, 0.75 ml of homogenate was precipitated with the same volume of 4% sulfosalicylic acid, centrifuged and mixed with 0.01 M 5,5-dithiobis-(2-nitrobenzoic) acid at ration 1:9. The color was immediately measured using at 412 nm. Results were expressed as nanomoles of GSH/mg protein. Superoxide SOD activity was tested as described [26]. In summary, the reaction was initiated by the addition of hydroxylamine HCl to the mixture containing nitroblue tetrazolium and the homogenate. The reduction was measured at 560 nm. The results were expressed as units/mg of protein [27].
2.6. Organs Weight
All the mice were sacrificed at the end of experiments and the weight of individual organs were evaluated.
2.7. Water-immersion Stress
Mice were forced to swim individually in a glass jar (30 cm x 12 cm x 30 cm) containing 18 cm-deep water at room temperature. After an initial period of vigorous activity each animal assumed a typical immobile posture. The mouse was considered to be immobile when it ceased to struggle and made minimal movements to keep its head above the water. This period of observation was 10 minutes.
2.8. Rotarod
The protocol is based on a study [28]. Mice were tested on the rotarod before the glucan treatment and after 1, 3 and 6 weeks into the trial. Mice were placed on the rotarod (755 Rotarod; IITC Life Sciences, Wooland Hills, GA, USA) at a speed starting at 3 rpm and gradually accelerating to 40 rpm over a 5 min period. The maximum trial length was 5 minutes and there was a 45 min recovery period between trials. An individual
Group | Body | Spleen | Thymus | Heart | Lungs |
---|---|---|---|---|---|
Control | 20.7 ± 1.8 | 601 ± 80 | 46 ± 10 | 118 ± 14 | 146 ± 17 |
PBS | 19.3 ± 2.2 | 329 ± 54 | 47 ± 12 | 126 ± 11 | 129 ± 14 |
Glucan 50 mg | 20.7 ± 1.7 | 479 ± 44* | 47 ± 9 | 101 ± 12 | 133 ± 15 |
Glucan 100 mg | 21.0 ± 1.8 | 572 ± 33* | 48 ± 11 | 120 ± 13 | 140 ± 10 |
Glucan 200 mg | 22.1 ± 1.8 | 602 ± 45* | 46 ± 12 | 118 ± 11 | 145 ± 9 |
average over the three trials was first calculated before finding a total group average.
3. RESULTS
Forced exercise did not result in significant changes of total body weight or weight of individual organs, with the exception of spleen weight. The strong reduction of spleen weight was restored by all doses of glucan (Table 1). Induction of fatigue by forced swimming for 6 minutes for 15 days increased the immobility period in animals supplemented with PBS (control group) and this immobility was observed in subsequent 30 days. All three doses of glucan significantly reduced the immobility periods, with the 50 μg dose being the least active (Fig. 1). The mice were subjected to daily swimming during all 45 days.
Levels of GSH and SOD were measured 15 days after start of the supplementation (30 days after start of the swimming). In both cases, glucan supplementation reversed the fatigue-caused decrease of these levels. The levels of GSH before the start of the experiment were 105 ± 1.9 and the levels of SOD were 97 ± 5.8. The effects of glucan were significant at 100 and 200 μg dose (Fig. 2).
Glucans are known to significantly affect various facets of the immune response. To find out the possible effects of glucan supplementation in our model, the changes in phagocytic activity were tested. Data summarized in Fig. (3) showed that all three doses of glucan significantly improved the suppressed phagocytic activity of peripheral blood neutrophils (control values were 30.3 ± 1.4).
In addition to these effects on cellular immunity, the effects on production of several important cytokines were studied. The depression of IL-2 formation (control level 255.5 ± 20.6 pg/ml) and elevation of IL-10 secretion (control level 21.4 ± 2.2 pg/ml) were found. In case of IL-4 formation, the control levels were 7.2 ± 0.6 pg/ml), therefore, the exercise-induced changes were not strong. In all cases, glucan supplementation returned the cytokines levels to normal values (Fig. 4).
In the last part of our study, the possible effects of glucan supplementation on motor functioning were focused. Using a rotarod test, we measured the average time to fall of mice subjected to a gradually accelerating rotarod before treatment, and after 1, 3 and 67 weeks of glucan supplementation. All glucan-supplemented groups showed significant improvements of motor functioning (Fig. 5).
4. DISCUSSION
β-Glucans belong to dietary fibers composed of D-glucose monomers linked by glycosidic bonds. They are commonly found in the cell walls of bacteria, algae, fungi and yeast. Their physiological, biological and physicochemical properties strongly differ depending on the source and isolation methods [29]. Health benefits of β-glucans have been extensively documented over last decades, resulting in its use both as a natural food supplements and, in some countries, as a drug [5, 30]. Among various biological effects of β-glucan supplementation, stimulation of immunity is the most pronounced. However, additional effects include lowering blood cholesterol [31], antioxidative effects [32], anti-allergic effects [33] and reduction of blood sugar levels [34]. So far, no adverse human effects have been reported.
Lately, β-glucans are being evaluated as a nutritional booster in various types of fatigue. In athletes, severe physical activity is known to negatively influence the immune functions and to increase the incidence of some infections. β-Glucan supplementation was documented to have positive effects on these problems, probably due to the changes in cytokine production [19].
Fatigue is one of the most common syndromes accompanying cancer disease. It can be caused by physical or mental stress and lead to a diagnosis of chronic fatigue syndrome. Over 50% of cancer patients describe that fatigue lasts for more than 12 months after the end of the treatment. This problem represents a multifactorial process involving several physiological and psychosocial factors [35]. Persisting fatigue is often connected with various immune processes including IL-6 production [36] and interferon and TNF-α release [35]. Glucan effects on TNF-α secretion is one of the anti-inflammatory effects of glucan [36-38]. In our clinical trial, significant anti-inflammatory effects of glucan supplementation in cancer-related fatigue were observed. [39]. In addition, numerous studies published lately showed that glucan improved exercise performance [40], improved syndromes of chronic fatigue syndrome via improved levels of GSH and SOD in the brain [41] and enhanced the endurance capacity while improving the fatigue-related recovery [41, 42]. The possible mechanisms suggested by these studies are improvements in oxidation and regulation of energy metabolism by glucan.
In this study, it has been identified that fatigued mice treated with glucan (50, 100, and 200 μg/day) orally had improved immune reactions depressed by fatigue. In addition, swimming times were improved by glucan treatment similarly to rotarod performance test. In our previous study, it has been found that short term supplementation help to improve fatigue-related problems [21]. In this study optimal dose and longer intervals of supplementation were focused. It was observed that even a relatively low 50 μg helped to improve tested reactions; the higher dose was found to be more active. In some reactions, the improvement was clearly dose-dependent. In addition to deregulated balance of Th1/Th2 related cytokines, significant changes in levels of SOD and GSH in the brain were found, indicating changes in oxidative stress in the glucan effects. Long-term treatment with glucan resulted in return to normal values.
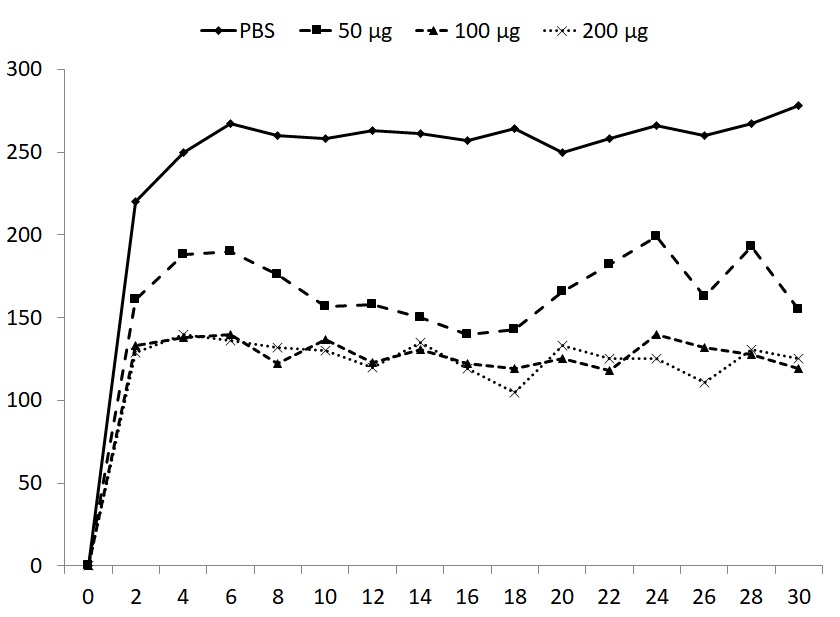
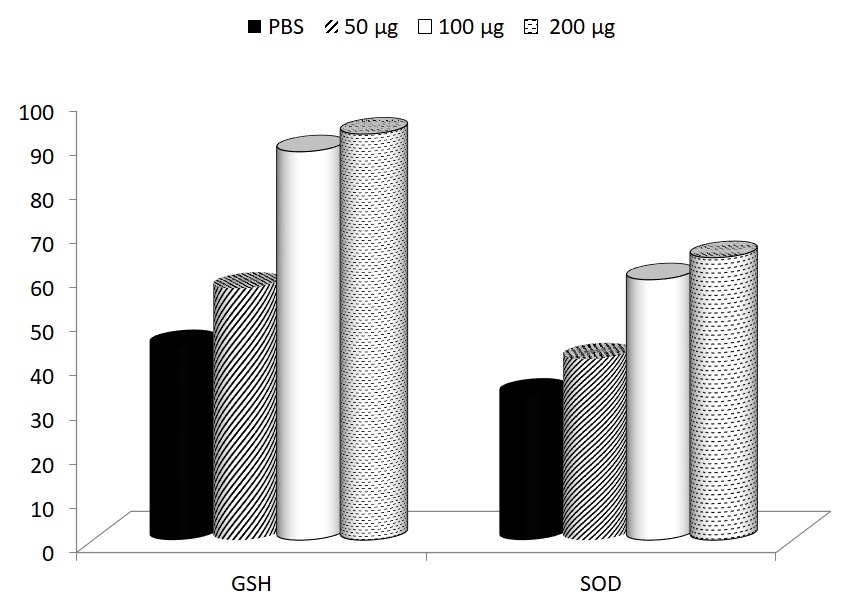
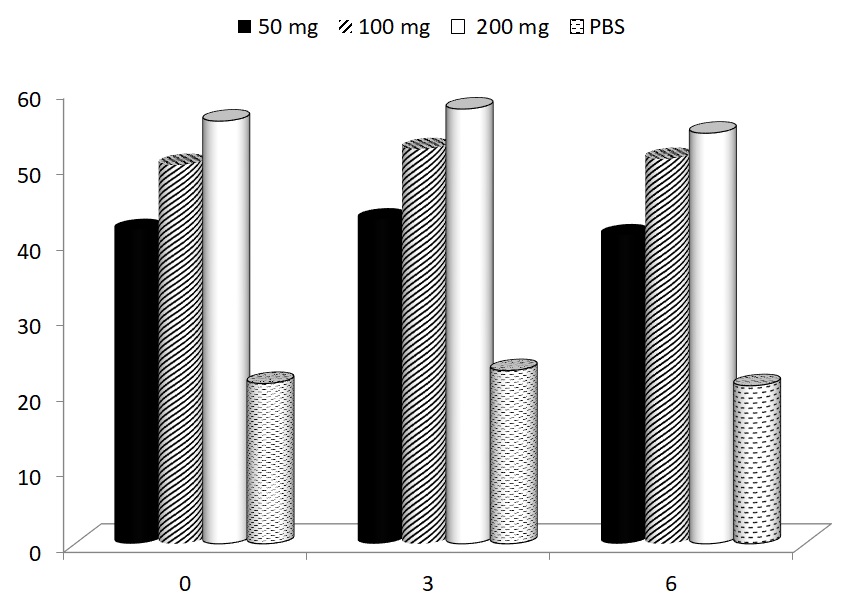
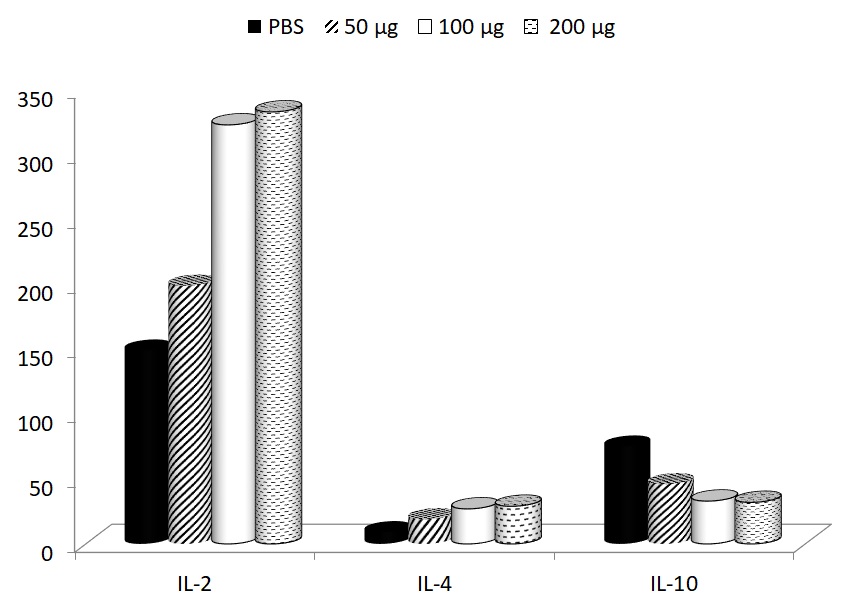
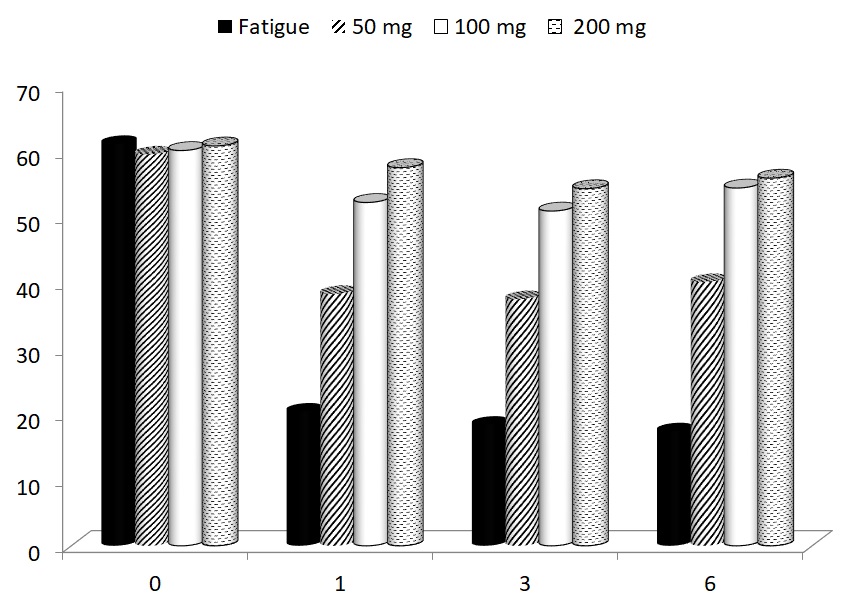
CONCLUSION
Although fatigue and possible use of natural molecules has received considerable attention lately [39], underlying mechanisms are still unclear. Our data suggested that anti-fatigue properties of glucan are related to its well-established effects as stimulator of immune reactions [37] and that this supplementation might offer an effective and safe treatment of problems associated with chronic fatigue. We believe that these results should encourage further studies into the potential use of glucan for the treatment of physical and/or mental fatigue.
ETHIC APPROVAL AND CONSENT TO PARTICIPATE
This study was approved by the Review Board of the University of Louisville, USA with approval number 18323.
HUMAN AND ANIMAL RIGHTS
No humans were used in the study. The research was conducted in accordance with the US Public Health Service's "Policy on Use of Laboratory Animals," and "Guide for the Care and Use of Laboratory Animals.".
CONSENT FOR PUBLICATION
Not applicable.
AVAILABILITY OF DATA AND MATERIALS
The authors confirm that the data supporting the findings of this research are available within the article.
FUNDING
None.
CONFLICT OF INTEREST
The authors declare no conflict of interest, financial or otherwise.
ACKNOWLEDGEMENTS
Declared none.