Effects of Triton X-100 and PEG on the Catalytic Properties and Thermal Stability of Lipase from Candida Rugosa Free and Immobilized on Glyoxyl-Agarose
Abstract
Background:
Candida rugosa Lipase (CRL) shows a very low alkaline stability that comprises its immobilization on glyoxyl-agarose, which requires pH above 10. In this way, an adaptation from the original method was used; an enzyme solution at pH 7 was slowly added at a suspension of glyoxyl-agarose prepared in bicarbonate buffer, pH 10. This change of protocol was enough for allowing the preparation of derivatives actives of CRL on glyoxyl-agarose and verifying the effect of this modified procedure on the properties of the immobilized enzyme. The effect of the additives Triton-X-100 and polyethylene glycol (PEG) on the enzymatic activity recovery and immobilized enzyme stability was evaluated.
Methods:
The glyoxyl-agarose support was prepared by etherification of 6% agarose beads with glycidol and further oxidation with sodium periodate. CRL was immobilized covalently on glyoxyl-agarose support in the absence and presence of 1% (w/v) Triton-X-100 or 5 g L-1 polyethylene glycol (PEG). The lipolysis activity of the free and immobilized enzyme was determined at 37ºC and pH 7.0, using p-nitrophenyl palmitate (p-NPP) as substrate. Profiles of temperature-activity (37-65ºC, pH 7.0) and pH-activity (6.0-9.5, 37ºC) were evaluated as well as thermal (45ºC and pH 8.0) and operational (15 min batches of p-NPP hydrolysis at 50ºC and pH 8.0) stabilities of free and immobilized CRL.
Results:
Using a single modification of the original protocol, the CRL poorly stable under alkaline conditions could be immobilized on glyoxyl-agarose in its active conformation (recovered activity varying from 10.3 to 30.4%). Besides, the presence of a detergent (Triton-X-100) and an enzyme stabilizer (PEG) contributed to the preparation of more active and more stable biocatalysts, respectively. CRL immobilized on glyoxyl-agarose in the presence of PEG was around 5 times more stable than the free CRL and around 3 times more stable than the CRL immobilized on glyoxyl-agarose in absence of PEG. The higher stability of the CRL-glyoxyl derivative prepared in the presence of PEG allowed its reuse in four successive 15 min-batches of p-nitrophenyl palmitate hydrolysis at 50ºC and pH 8.0.
Conclusion:
The technique of immobilizing enzymes covalently on glyoxyl-agarose showed promising results for Candida rugosa lipase (CRL). The derivatives prepared in the presence of the additives retained two to three times more activity than those prepared in the absence of additives. The enzyme immobilized in presence of PEG was about three times more stable than the enzyme immobilized in absence of this additive. Maximum catalytic activity of the immobilized CRL (in absence of additives) was observed in a temperature 10ºC above that for the free enzyme and the pH of the maximum activity was maintained in the range 6.5-7.5 for free and immobilized CRL.
1. INTRODUCTION
Lipases (E.C. 3.1.1.3) are hydrolytic enzymes that catalyze the hydrolysis of triglycerides to produce mono and diacylglycerides, free fatty acids and glycerol. However, when water activity in the reaction medium is low, the majority of lipases can exert their reverse enzymatic activity, i.e., catalysis of esterification, transesterification (e.g., acidolysis, alcoholysis, and aminolysis), and interesterification reactions [1-8]. Because of this great catalytic versatility and their high stability in organic and aqueous medium, lipases currently have gained significant share of the world enzyme market.
In general, lipases act at a wide pH range, are stable at high temperatures and do not require cofactors. In addition, they exhibit high specificity and properties of regio-, chemo- and enantioselectivity [9, 10]. These enzymes are the most important among hydrolases due to their multiple applications in industrial processes. Lipases are used in the detergents, medicines, food, textiles, pulp and paper, cosmetics, biodiesel and biosensors industries, as well as applied in the treatment of effluents [10, 11].
Lipases show a typical mechanism of action called interfacial activation [12]. In aqueous media the active site is protected from the reaction medium by an oligopeptide chain known as “lid”, which in the closed form turns the enzyme inactive [13]. The closed form of the lipase (closed lid) may exist in equilibrium with an open form, where the lid has been unlocked, allowing the access of the substrate to the active site [14]. When the enzyme is exposed to a hydrophobic interface (drops of oil, hydrophobic support surfaces, gas bubbles, hydrophobic proteins, lipopolysaccharides, etc), the lid is opened exposing hydrophobic residues located in the internal face of the lid and in the surroundings of the active site, shifting the equilibrium toward the open enzyme structure [12, 13, 15-18]. It have been reported that the presence of detergents in the lipase medium also favors the shifting of the equilibrium towards to the open lid form, allowing the fixation of the lipase on hydrophobic supports in a hyperactivated conformation [19-26]. Besides, lipases may exist in solution as biomolecular aggregates, in which the hydrophobic active centers of the monomers are in close contact, yielding more stable and less active structures. In presence of detergents, these aggregates are dissociated yielding less stable and more active monomers, which may be fixed on glyoxyl supports with the active center turned to the reaction medium [23].
In the last years, studies of lipase immobilization have increased considerably aiming their application in different fields, such as, hydrolysis of oils and fats, and esterification reactions for producing chemical compounds for food, cosmetic and nutraceutical industries [27-29]. Lipases from different microorganisms have been immobilized on various activated supports, such as, octyl-agarose, octadecyl-Sepabeads, DEAE-Sepharose, IDA-Cu+2-Sepabeads, MANAE-agarose, CNBr-Sepharose and glyoxyl-agarose [30-40].
Among the great number of activated supports available for immobilizing enzymes [41], active and stable derivatives of many enzymes have been produced using glyoxyl-agarose as support, such as Penicillin G acylase [42, 43], trypsin [44], chymotrypsin [45], thermophilic esterase [46], L-aminoacylase [47], chitosanase [48], carboxypeptiase A [49, 50], alcalase [51], cyclomaltodextrin glucanotransferase [52, 53] and lipase [23, 37, 54, 55].
Glyoxyl-agarose is composed of thick agarose fibers that hold a large number of very stable aldehyde groups linked to the support by very short spacer arms. Under alkaline conditions (pH around 10.0), enzyme immobilization occurs through, at least, a two-point reaction between the protein surface region having higher densities of amino groups and the support aldehyde groups. A more intense multipoint attachment between the immobilized protein and the activated support can be reached, with minimal loss of catalytic activity, by a long-term incubation of the enzyme–support reaction medium under suitable conditions, such as temperature around 20-25ºC. The last step of the immobilization of an enzyme on glyoxyl-agarose is a very mild reduction using sodium borohydride. After that, the enzyme remains attached to the support by means of very stable secondary amino bonds (with physical properties very similar to the initial primary amines) and the non reacted aldehyde groups on the support are transformed into hydrophilic hydroxyl groups [21].
Palomo et al. [33] reported that lipase from Candida rugosa could not be immobilized on glyoxyl-agarose by the original protocol [45], which requires the suspension of the support in an enzyme solution at pH 10. However, in this work, a simple modification of the original method allowed the immobilization of this lipase in this support: an enzyme solution at pH 7 was slowly added in a suspension of glyoxyl-agarose prepared in bicarbonate buffer 80 mM, pH 10. Besides, CRL was also immobilized on glyoxyl-agarose in the presence of Triton-X-100 (a surfactant that may activate lipases and dissociate bimolecular aggregates) or polyethylene glycol (a stabilizer of enzymes) and their influence on the enzymatic activity recovery and thermal stability of the immobilized enzyme was evaluated.
2. MATERIALS AND METHOD
2.1. Materials
Lipase from Candida rugosa (type VII), glycidol (2,3-epoxy-1-propanol), p-nitrophenyl palmitate (p-NPP) and sodium borohydride were acquired from Sigma Chem. Co (St. Louis, MO, EUA). Sepharose CL-6B was purchased from Amersham Pharmacia Biotech AB (Uppsala, Sweden). Sodium metaperiodate, Triton X-100, gum arabic and polyethylene glycol (PEG- 1450) were purchased from Synth (Diadema, SP, Brazil). All other chemicals were of analytical grade.
2.2. Preparation of CRL Glyoxyl-Agarose
2.2.1. Support Activation
The glyoxyl-agarose support with high concentration of aldehyde groups per volume (around 75 µmol mL-1) was prepared by etherification of 6% agarose beads with glycidol and further oxidation with sodium periodate [42].
Under gentle stirring and in an ice bath, 105 g of agarose, previously washed with distilled water, were mixed with 30 mL of distilled water, and 50 mL of 1.7 N NaOH, containing 1.425 g sodium borohydride (previously cold prepared). After, 36 mL of glycidol were added dropwise to avoid raising the temperature above 25ºC, and the suspension formed was gently stirred for 12-15 h. The etherified support (glyceryl-agarose) was washed with distilled water, dried by vacuum suction in a sintered glass filter, and resuspended in 895 mL of water (volume ratio support:suspension of 1:10). Sodium metaperiodate (3.21 g) was added and the suspension was gently stirred for 2 h at room temperature. Finally, the glyoxyl-agarose support was washed with water, filtered under vacuum and suck dried.
2.2.2. Immobilization of Enzymes on Glyoxyl-Supports
A mass of 10 g of glyoxyl-agarose were added to 90 mL of 80 mM sodium bicarbonate buffer, pH 10 and maintained under gentle agitation at 25ºC. Next, 10 mL of enzyme solution (0.97 to 4.87 mg of protein/mL) prepared in 50 mM sodium phosphate buffer, pH 7.0, were slowly added. Then, this immobilization suspension was kept under gentle agitation at 25ºC for two or four hours. The enzymatic activity of the initial solution and the final supernatant obtained after the immobilization period was measured to determine the amount of enzyme fixed in the support. After 4 h reaction time, no enzymatic activity was detected in the supernatant and the immobilized lipase derivative was reduced with sodium borohydride (1 g L-1, 25ºC, 30 min), as described by Guisán and Blanco [44]. Then, the CRL-derivative was washed with excess distilled water, filtered under vacuum, and suck dried.
CRL was also immobilized in the presence of 1% (w/v) Triton-X-100 [56] or 5 gL-1 polyethylene glycol (PEG).
All experiments were performed at least in triplicate and the standard deviations were less than 10%.
2.3. Activity Assay
The lipolytic activity of the free and immobilized enzyme was determined at 37ºC, pH 7.0, using p-nitrophenyl palmitate (p-NPP) as substrate [57]. An emulsion of 0.8 mM p-NPP was prepared by mixing 1 mL of p-NPP solution (3 gL-1 in isopropanol) and 9 mL of 50 mM phosphate buffer at pH 7.0 containing Triton X-100 and gum arabic (approximately 0.45 and 1.11 gL-1, respectively).
For the free CRL, 0.3 mL of an enzyme solution (protein concentration equal to 9.74 µg mL-1) was mixed with 2.7 mL of substrate emulsion in a 1 cm optical length cuvette and the absorbance change at 37oC was monitored for 10 min at 410 nm in a UV-1203 spectrophotometer (Shimadzu Co., Japan), using pure substrate emulsion as reference. For the immobilized CRL, a mass of approximately 1 mg of immobilized CRL was suspended in 30 mL of substrate emulsion in a batch reactor thermostatized at 37oC and samples were withdrawn at regular time intervals up to 10 min reaction for measuring the absorbance change at 410 nm in a UV-1203 spectrophotometer (Shimadzu Co., Japan), using pure substrate emulsion as reference.
The enzymatic activity per mass of enzyme (U g-1) was calculated with Equation 1:
![]() |
(1) |
where ΔABS is the reaction medium absorbance change after 10 min reaction time (cuvette of 1 cm optical length); VR is the total reaction volume (3 mL for the free enzyme and 30 mL for the immobilized enzyme), ε is molar extinction coefficient for the p-NPP (ε = 8.03 x 103 M-1 cm-1) and mE is the free or immobilized enzyme mass in g. Factor 103 was required for unit conversion (mol of p-nitrophenol to μmol of p-nitrophenol).
One Unit of enzymatic activity (U) was defined as the amount of enzyme that releases 1 μmol of p-nitrophenol per min under the experimental conditions describe above. Under these conditions the free CRL activity was 47.6 ± 3.6 U mg-1 of protein. The free enzyme protein content was 32.47 mg g-1 as determined by the Bradford’s method [58].
2.4. Temperature-activity and pH-activity Profiles
Enzymatic activities of free and immobilized CRL were measured at 37-65ºC (pH 7.0) and at pH 6.5-9.5 (37ºC), using p-NPP as substrate, using the activity method described in the Section 2.3. The molar extinction coefficients (M-1 cm-1) at different pH were calculated for measurements of activity.
2.5. Thermal and Operational Stability
The thermal stability of the CRL-agarose biocatalysts was evaluated at 45ºC and pH 8.0. Biocatalyst suspensions were prepared in 50 mM sodium phosphate buffer, containing Triton X-100 and gum arabic at the concentrations of 0.45 and 1.11 gL-1, respectively. Residual activity was measured in 30 min intervals adding 0.8 mM p-NPP solution prepared in isopropanol. After 15 min at 37ºC the absorbance was measured at 410 nm.
The parameters of thermal inactivation were estimated by fitting the model of Sadana and Henley [59] to the experimental data of residual activities vs. time (Eq. 2):
![]() |
(2) |
where A/A0 is the dimensionless residual activity, α1 is the ratio of the specific activity of the final state to the initial state, and k1 is the first-order deactivation rate constant (time-1).
The two-parameter model was fitted to the deactivation data using the Levenberg-Marquardt method of iterative convergence, at 0.95 confidence level. The catalyst half-life (t½) was calculated using Eq. 3:
![]() |
(3) |
The operational stability of CRL-glyoxyl agarose derivatives prepared in the absence and presence of PEG was also evaluated at 50oC, pH 8.0 in reaction medium containing 1 mL of p-NPP (3 gL-1 in isopropanol) and 9 mL of 50 mM phosphate buffer, pH 8.0 containing Triton-X-100 (0.45 gL-1) and gum Arabic (1.11 gL-1). The residual activities of the biocatalysts were assessed in 15 min-intervals using the procedure described in section 2.3.
3. RESULTS AND DISCUSSION
3.1. Immobilization of CRL on Glyoxyl-Agarose
All CRL-derivatives prepared according to Section 2.2.2 achieved 100% immobilization yield, i.e., all protein offered to the support (1 or 5 mg g-1) was immobilized.
Table 1 shows great losses of enzyme activity (65-87%) for CRL immobilized on glyoxyl-agarose in absence or presence of Triton-X-100 or PEG. These losses are probably associated to the low enzyme stability at alkaline pH [33]. In fact, the immobilization of CRL according to the original protocol [42], in absence or presence of additives, resulted in biocatalysts completely inactivated. On the other hand, the slowly enzyme addition was sufficient for allowing the preparation of active CRL derivatives.
Additive | Enzyme Activity Offered per Mass of Support (U g-1) |
IEb Activity per Mass of Support (U g-1) |
Activity Recoveryd (%) |
---|---|---|---|
Absencea | 46.2 | 5.8 | 12.6 |
Triton-X-100 (1%, w/v)c |
231.8 | 82.1 | 35.4 |
PEG (g L-1)c | 231.8 | 58.2 | 25.1 |
The use of Triton-X-100 (1%, w/v) yielded recovered activity higher than that obtained with PEG (5 gL-1), which may be associated with the dissociation of bimolecular aggregates (more stable and less active), allowing the immobilization of CRL molecules on glyoxyl-agarose as monomers (less stable and more active) [23]. It have been reported that the addition of a detergent under the critical micellar concentration may increase the lipase activity by shifting the conformational equilibrium of lipases toward the open form. However, detergents may also have negative effects on enzyme activity above a certain concentration [60].
The stabilizing effect of additives on the activity of an enzyme, such as sugars and polyols (e.g., PEG), is based on the reduction of the dielectric constant of the solution compared to the pure water. Consequently, dielectric interactions, as well as hydrophobic ones, between enzyme functional groups should be stronger, making the enzyme molecule more rigid and thus more thermally stable [61]. This effect may have contributed to preserve the CRL activity in alkaline pH, but without allowing the immobilization of the enzyme in the monomeric and more active form because of the greater rigidity of the molecule in the presence of PEG impairing the bimolecular aggregate dissociation. This may explain the lower activity recovered when PEG was used as additive instead Triton-X-100 for CRL immobilization.
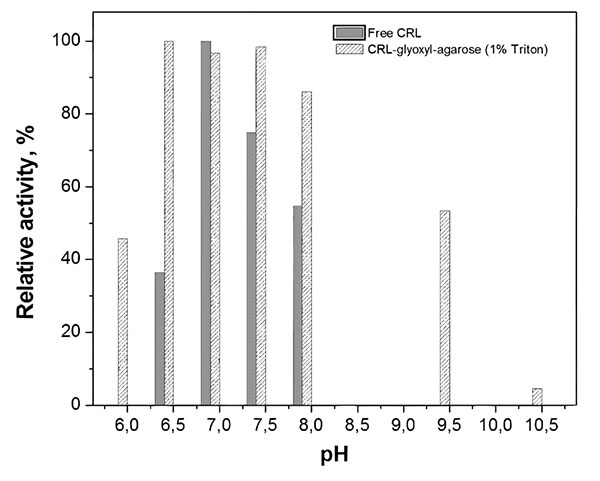
3.2. Profiles of Activity vs pH and Temperature
The activity-pH profile for free and immobilized CRL was evaluated in the range 6.0-10.5. Fig. (1) shows that the pH of maximum activity was 7.0 for free CRL and 6.5-7.5 for immobilized CRL in the presence of Triton-X-100 (1%, w/v), which is consistent with the fact that glyoxyl-agarose is a non-charged support, therefore not changing the microenvironment closed to the immobilized lipase [62]. Similar behavior was also reported by Pereira et al. [63] for CRL immobilized on chitosan and by Soares et al. [64] for CRL encapsulated into hydrophobic sol-gel matrix.
The catalytic activity for free and immobilized CRL was measured at pH 7.0 in the temperature range of 37-60ºC. Fig. (2) shows that for immobilized lipases the temperature of maximum catalytic activity occurs between 45 and 50ºC (except for CRL immobilized in presence of PEG), while for free CRL this maximum is found approximately at 40ºC. Similar behavior has been previously reported [63-66]. The 5-10ºC raise in the maximum activity temperature for immobilized CRL demonstrates its greater stability as consequence of the 3D-structure rigidification promoted probably by the formation of multiple covalent links enzyme-support.
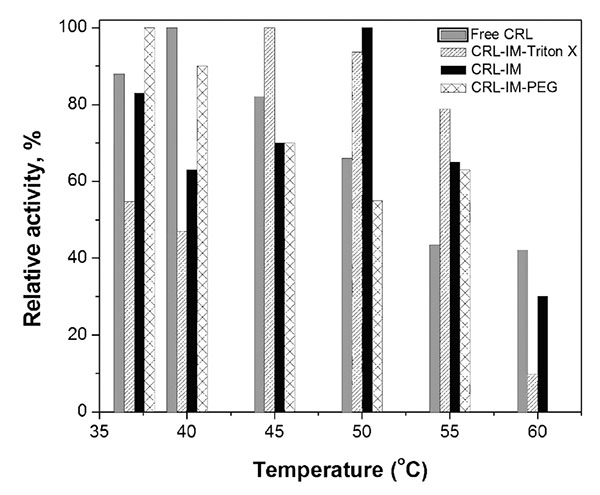
3.3. Thermal and Operational Stability
Fig. (3) shows the inactivation profiles of free and immobilized CRL at 45ºC and pH 8.0 during a time period of 120 min. CRL free and immobilized on glyoxyl-agarose (presence of Triton-X-100) showed similar behavior of inactivation, rapidly dropping its initial activity to around 30% after 30 min incubation. On the other hand, in same time interval, CRL immobilized in the presence of PEG retained around 95% of its initial activity.
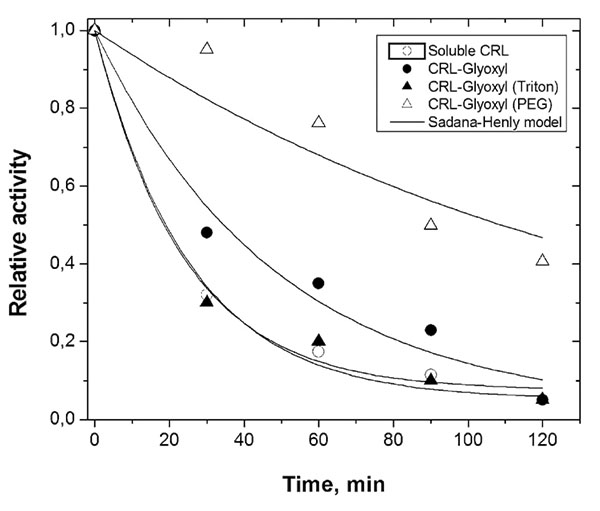
The half-lives of CRL immobilized on glyoxyl-agarose in the absence and presence of PEG were estimated as 34.5 and 109 min, respectively, representing a stabilization factor around 3 times. Compared to the free CRL (half-life around 18.5 min), the CRL immobilized on glyoxyl-agarose in presence of PEG showed to be around 6 times more stable.
Probably, CRL was immobilized on glyoxyl-agarose (absence and presence of PEG) as bimolecular aggregates (more stable and less active) and CRL was immobilized on glyoxyl-agarose (presence of Triton-X-100) as monomers (more active and less stable).
The operational stability is a parameter of fundamental importance when it is desired to use the immobilized enzyme industrially [67]. Therefore, the stability of the immobilized biocatalysts prepared in the absence and presence of PEG (the most thermally stable biocatalysts) were evaluated in the reaction medium of p-NPP hydrolysis at 50ºC and pH 8.0. Fig. (4) shows that CRL immobilized on glyoxyl-agarose in the presence of PEG was around 3 times more stable than the one immobilized in absence of PEG. This result shows the stabilizing effect of PEG, allowing the immobilization of CRL on glyoxyl-agarose in a rigid and stable conformation.
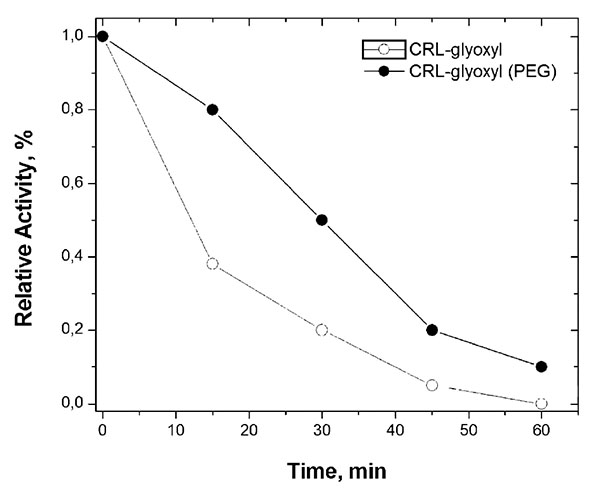
CONCLUSION
The technique of immobilizing enzymes covalently on glyoxyl-agarose showed promising results for Candida rugosa lipase (CRL). Using a single modification of the original protocol, i.e., adding slowly the enzyme solution prepared at pH 7.0 in the support suspension prepared at pH 10.0 instead of preparing the enzyme solution directly at pH 10.0, the CRL poorly stable under alkaline conditions could be immobilized on glyoxyl-agarose in its active conformation (recovered activity varying from 10.3 to 30.4%). Besides, the presence of a detergent (Triton-X-100) and an enzyme stabilizer (PEG) contributed to the preparation of more active and more stable biocatalysts, respectively. CRL immobilized on glyoxyl-agarose in the presence of PEG was around 5 times more stable than the free CRL and around 3 times more stable than the CRL immobilized on glyoxyl-agarose in absence of PEG. The higher stability of the CRL-glyoxyl derivative prepared in the presence of PEG allowed its reuse in four successive 15 min-batches of p-nitrophenyl palmitate hydrolysis at 50ºC and pH 8.0.
ETHICS APPROVAL AND CONSENT TO PARTICIPATE
Not applicable.
HUMAN AND ANIMAL RIGHTS
No Animals/Humans were used for studies that are base of this research.
CONSENT FOR PUBLICATION
Not applicable.
CONFLICT OF INTEREST
The authors declare no conflict of interest, financial or otherwise.
ACKNOWLEDGEMENTS
The authors thank National Counsel of Technological and Scientific Development (CNPq), Coordination for the Improvement of Higher Education Personnel (CAPES) and Araucária Foundation for the financial support.
REFERENCES
[PubMed Link]
[PubMed Link]
[PubMed Link]
[PubMed Link]
[PubMed Link]
[PubMed Link]
[PubMed Link]
[PubMed Link]
[PubMed Link]
[PubMed Link]
[PubMed Link]
[PubMed Link]
[PubMed Link]
[PubMed Link]
[PubMed Link]
[PubMed Link]
[PubMed Link]
[PubMed Link]
[PubMed Link]
[PubMed Link]
[PubMed Link]
[PubMed Link]
[PubMed Link]
[PubMed Link]
[PubMed Link]
[PubMed Link]
[PubMed Link]
[PubMed Link]
[PubMed Link]
[PubMed Link]