All published articles of this journal are available on ScienceDirect.
Nitric Oxide and its Metabolites in the Critical Phase of Illness: Rapid Biomarkers in the Making
Abstract
The potential of nitric oxide (NO) as a rapid assay biomarker, one that could provide a quantum leap in acute care, remains largely untapped. NO plays a crucial role as bronchodilator, vasodilator and inflammatory mediator. The main objective of this review is to demonstrate how NO is a molecule of heavy interest in various acute disease states along the emergency department and critical care spectrum: respiratory infections, central nervous system infections, asthma, acute kidney injury, sepsis, septic shock, and myocardial ischemia, to name just a few. We discuss how NO and its oxidative metabolites, nitrite and nitrate, are readily detectable in several body compartments and fluids, and as such they are associated with many of the pathophysiological processes mentioned above. With methods such as high performance liquid chromatography and chemiluminescence these entities are relatively easy and inexpensive to analyze. Emphasis is placed on diagnostic rapidity, as this relates directly to quality of care in acute care situations. Further, a rationale is provided for more bench, translational and clinical research in the field of NO biomarkers for such settings. Developing standard protocols for the aforementioned disease states, centered on concentrations of NO and its metabolites, can prove to revolutionize diagnostics and prognostication along a spectrum of clinical care. We present a strong case for developing these biomarkers more as point-of-care assays with potential of color gradient test strips for rapid screening of disease entities in acute care and beyond. This will be relevant to global health.
INTRODUCTION
The emergency department (ED), closely linked to the critical care setting, is widely used as first and last resort for acute as well as chronic disease management. In fact, with the advent of the economic recession in the U.S. and concomitant loss of health insurance in families, usage of the ED has increased tremendously [1, 2]. The ED and critical care settings provide a unique clinical venue to develop, test and implement novel biomarkers that can be cost-effective and rapid in their ability to manage acute as well as chronic disease processes with acute exacerbations. Much of the current laboratory testing is cost and time inefficient and presents detectable amounts of the biomarker only in the later periods of the pathological cycle. For example, the most prevalent traditional biomarker of renal dysfunction and the gold-standard, serum creatinine, can take up to 72 hours to rise in early acute renal failure (acute kidney injury); baseline levels may be unavailable, thus it renders itself as a biomarker with limited utility in the acute setting [3, 4]. There is an unmet need for viable and rapid biomarkers for various acute disease states, such as bronchiolitis, asthma, influenza-like illness (with increased numbers during pandemics), sepsis, shock, cardiovascular-related acute ischemia / injury in the coronary and cerebral vasculature, to name a few. Thus, these novel biomarkers can potentially enhance disease management in a variety of clinical settings.
Nitric oxide (NO) and its metabolites, as potential signaling molecules, are implicated in a wide variety of physiological and pathological states. This review will cover some of the more recent progress made in establishing the utility, and limitations, of NO and its metabolites, nitrite and nitrate, in a few acute disease states. The utility of these will be extrapolated to disease processes beyond the ED, i.e., to the critical care setting, along a continuum of care.
NITRIC OXIDE AND ITS METABOLITES: PHYSIOLOGICAL AND BIOCHEMICAL ASPECTS
NO as a highly reactive gaseous signaling molecule and free radical is implicated in a variety of physiological and biochemical processes (Fig. 1). Although it has been associated with virtually every organ system in the body, it’s most important roles are 3 fold: regulation of blood flow in the vascular endothelium (vascular homeostasis), neurotran-smission and host defense mechanisms [5].
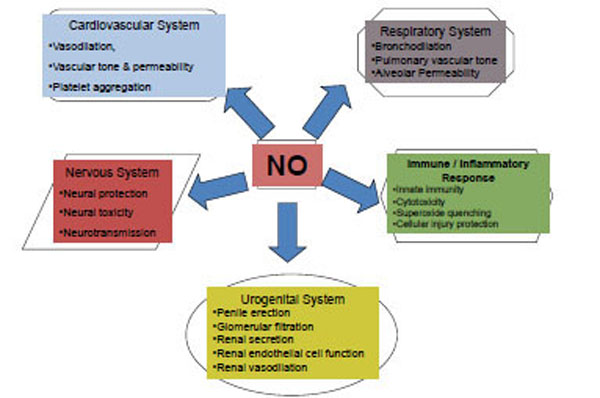
Nitric oxide (NO): Diversity in action.
The predecessor to part of NO produced in the body is the semi-essential amino acid L-arginine (Fig. 2). NO production is catalyzed by 3 isoforms of NO synthase (NOS): endothelial or eNOS by vascular endothelium in the cardiovascular system, neuronal or nNOS, primarily operational in producing NO as a neurotransmitter, and inducible or iNOS, produced by a variety of cells (endothelial and otherwise) for a variety of reasons, including an immune-mediated or inflammatory response [6]. Both the calcium dependent NOSs (eNOS and nNOS), have transient activities and thus exhibit relatively low concentrations, usually ranging in the nM range, while the cytokine activated, calcium independent, iNOS is long lasting, generating higher concentrations of NO, usually in the µM range [6]. As a vascular effector, NO rapidly diffuses from its site of generation in endothelial cells into adjacent smooth muscle cells, where it activates soluble guanylate cyclase (Fig. 2). The latter enzyme converts GTP to cGMP, the second messenger that affects vascular smooth muscle relaxation [7].
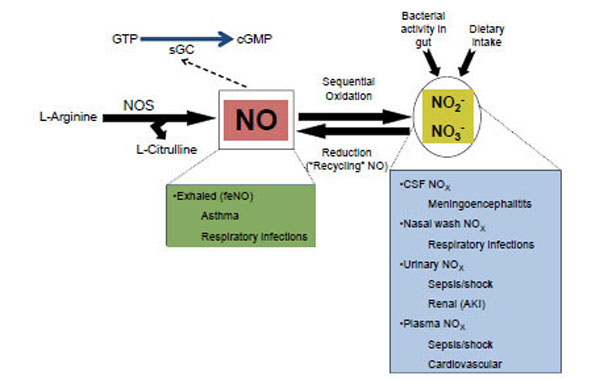
The nitric oxide (NO), nitrite (NO2-) and nitrate (NO3-) cycle. Formation of NO, oxidation to nitrite and nitrate (collectively, NOx), and their potential as biomarkers for disease processes in the acute care setting. Acute kidney injury (AKI); Cerebrospinal fluid (CSF); Central nervous system (CNS); Cyclic guanylate monophosphate (cGMP); Fractional excretion of nitric oxide (feNO); Guanylate triphosphate (GTP); Nitric oxide synthase (NOS); Soluble guanylate cyclase (sGC).
Once produced and active, NO can exist as the nitrosium cation (NO+), the nitroxyl anion (NO-), and a NO free radical (all with half lives < 20 s in circulation); all of these forms then undergo a sequential oxidation or nitrosation of water to form the more stable NO metabolites, nitrite [t1/2=110 s in blood] and nitrate [t1/2=5-8 h in blood] [8] (Fig. 2). Fig. (2) also shows that nitrate and nitrite (collectively referred to as NOx) can be recycled to produce NO (via reduction) in various ways, one of which is the enterosalivary circulation of endogenous nitrate, an essential pathway in maintenance of NO homeostasis [8-11]. NOx in blood as an index of eNOS activity has been widely used as routine indirect measure of NO levels [12-15]. Recent studies have indicated that nitrite is an important signaling molecule in its own right [16, 17]. Since it can be reduced back to NO given appropriate circumstances, this has led to intense interest in this relatively simple molecule [18]. A significant contribution of circulating levels of NOx is from dietary intake of those moieties, and these can vary tremendously from diet to diet [8]. During fasting conditions with low dietary intake of NOx, enzymatic formation of NO via NOS accounts for the majority of circulating NOx. Nitrate and nitrite have been shown to be biomarkers for cardiovascular and other diseases from both diagnostic and therapeutic aspects [6, 8]. In addition to blood, urinary levels of NOx provide a means to assess systemic production of NO in vivo, however, with the caveat that urinary (or plasma) levels are truly reflective of endogenous production of NO, dietary ingestion of NOx, as well as from bacterial activity in the gut [6, 8].
The interaction of NO and NOx with other moieties such as superoxide (to form peroxynitrite), thiols (to form S-nitrosothiols), secondary amines (to form N-nitrosoamines), and metals (to form nitrosyl-heme) is also important physiologically. As a free radical NO is bactericidal at baseline, however, it has the additional property of scavenging superoxide and by doing so protects against injury [6]. The double edged nature of this reaction though is evidenced by the resultant peroxynitrite, a potent cytotoxic agent, implicated in disease processes, and an area of intense research effort [19].
NO AND ITS METABOLITES IN ACUTE DISEASE PROCESSES
NO and its Metabolites as Markers of Ongoing Infection and Inflammation
In addition to the various physiological and biochemical properties and functions described above, NO can also be a biomarker for many inflammatory disease states, either local to the tissue where generated or systemically. The effects of NO as an inflammatory mediator have been studied in detail for a length of time. NOS and NO induction have been shown to be innate immune effectors of infectious/inflammatory pathways [20]. The precise effect that NO has on these disease states and inflammatory mediation is still under debate. A recent study showing the temporal effects of NO metabolites in a rodent model of systemic inflammation and sepsis revealed tissue specific and time specific changes in NO metabolites [21]. This highlights ‘the NO paradox’: is NO a helpful mediator in inflammatory disease, or does it function as a cytotoxic effector of non-specific immune response? In a ‘too much of a good thing’ scenario, during the immune response, moderate levels of NO prove beneficial to the organism, while excess levels lead to too much potency and thus damage.
NO and its Metabolites in Acute Respiratory Infections
Respiratory syncytial virus (RSV) and influenza can cause major respiratory infections. RSV is the most common cause of lower respiratory tract infections in children, including bronchiolitis and pneumonia [22, 23]. There is a broad spectrum of disease severity for bronchiolitis, the leading cause of hospitalization for infants in the U.S. with associated hospitalization costs in excess of $500 million per year, as well as responsible for frequent ED visits [24, 25]. Pandemics like the 2009 novel swine-origin influenza A (H1N1) virus (nH1N1) pose significant challenges: increased transmission relative to seasonal influenza, severe disease in patients with medical co-morbidities, and difficulty in predicting which patients with viral respiratory symptoms are at risk for influenza complications [26]. In the evaluation of acutely ill patients with respiratory infections, risk stratification may be achieved by utilizing biomarkers, augmenting clinical decision-making, thus improving outcomes. This is particularly relevant to the acute care setting.
During influenza virus infection, epithelial cells, macrophages, lymphocytes and other cells release a complex series of cytokines and chemokines in an attempt to overcome viral replication and disease progression [27-29]. NO, a key mediator of airway inflammation is also released. It is unclear if increased NO activity is a marker of clinical disease, of the innate antiviral response, or associated significantly with a unique respiratory virus infection [30]. In the murine model of RSV for instance, NO production in the lungs appears to be associated with viral clearance and lung disease [31]. iNOS activity and production of NO were associated with enhanced clearance of RSV, yet they were also associated with airway inflammatory changes and airway dysfunction [31]. In murine studies of experimental influenza A-triggered pneumonia, excessive NO biosynthesis, as measured by its metabolites nitrate and nitrite, was demonstrated in the lungs [32, 33]. This suggested that the metabolites may be markers of influenza-mediated disease in the respiratory tract.
In humans, NO is produced in the upper and lower respiratory tract. It can be detected in exhaled air of healthy individuals and at increased levels in subjects with pulmonary disease, including asthma (see below), bronchiectasis and upper respiratory infections [34-38]. In aqueous solutions, NO is rapidly converted to distinct oxides of nitrogen, which may thus be potential biomarkers of disease severity in acutely ill patients presenting with influenza-like-illness (ILI) [35, 39]. During the 2009 nH1N1 pandemic, we collected nasal wash specimens from children with acute ILI, and then measured NOx concentration in the nasal secretions by HPLC. Overall, our data suggested that concentration of NO-derived nitrate in nasal secretions in children may be related to type of viral pathogen causative for acute ILI: RSV subtype B-associated ILI had higher nasal wash nitrate compared to all other viruses combined (P=0.002) [40]. Ongoing studies will determine the predictive potential of this putative biomarker for important disease outcomes in sicker patients requiring critical care.
Exhaled NO has been studied as a putative biomarker for respiratory infections caused by influenza, rhinovirus and other viral pathogens, but the data have been equivocal [41, 42]. The conflicting data above may simply reflect technical issues in measuring exhaled NO. This methodology is cumbersome to achieve in the pediatric population, either in-patient or ED. The newer NIOX-MINO hand-held NO detector has proven to be as reliable as the older, stationary chemiluminescence analyzer, the Ecomedics (ECO) [43]. Its portability may make it easier to handle in acute care settings of the ED or critical care. Focusing on stable NO metabolites in nasal secretions from patients with acute ILI may still be the better approach because of ready accessibility. Future studies are needed that can establish correlations between nasal secretion NOx and exhaled NO for acute ILI either in the ED or critical care settings.
NO and its Metabolites in Acute Central Nervous System (CNS) Infections
Meningitis can be a severe infection of the CNS. Bacterial meningitis, in particular, remains an important public health problem worldwide, with several studies confirming the devastating neurological sequelae that may result from it [44]. Cases of varying severity are likely to pass through the ED; and the more severe ones then sent to critical care. Early cerebrospinal fluid (CSF)-based biomarkers are needed that may be able to predict severity, so that potentially sicker patients can then get critical care support. NO and its metabolites in the CSF may represent such a biomarker. Studies have shown that patients admitted to the hospital with bacterial meningitis and influenza-associated encephalopathy had higher levels of CSF NOx as compared to controls [45]. In another study, patients with RSV infection and symptoms of encephalitis, encephalopathy or seizures were found to have a higher concentration of CSF NOx compared with those with influenza positive encephalopathy or those without meningitis [46]. Neither were the studies set up in the ED nor did they analyze CSF NOx in meningitis from different pathogens in the same setting. Using a rapid HPLC-based assay for detection of these metabolites in CSF in the acute setting may add to the current armamentarium for helping in early diagnosis of different forms of meningitis and may be predictive of disease severity. Detecting CSF NOx in a rapid assay may be particularly helpful in cases that are difficult to interpret, such as in cases of CSF pleocytosis. The added information may help guide management, ultimately resulting in better disease outcomes.
NO in Acute Asthma Exacerbations
Asthma is a major public health issue [47]. Acute asthma exacerbations are common causes for pediatric and adult ED visits with significant morbidity and mortality associated with them [48]. Prompt diagnosis and treatment in the ED and beyond are needed. There are no definitive diagnostic and predictive tests for asthma, specifically in the acute setting. NO is thought to play an important role in the pathogenesis of asthma by modifying airway hyperreponsiveness and inflammation. Studies in asthmatic children and adults have shown that fractional excretion of nitric oxide (feNO) reflects the degree of airway inflammation, with higher levels correlating with severity of disease, likely secondary to inflammation. As such, it can be a non-invasive biomarker for severity of the disease process and response to treatment [49-51]. In several studies the adequacy of anti-inflammatory treatment for chronic asthma has been demonstrated by lowered concentration of feNO [52-55], indicating the potential of NO as a management tool for asthma. Based on this, Kwok et al. performed a cross-sectional study in children 2-18 years of age presenting to an urban ED with an acute asthma exacerbation [56]. Using a tidal breathing method and a chemiluminescence-based NO analyzer, they showed that only 68% of the subjects could provide adequate breaths for feNO measurements [56]. Furthermore they showed that this method had limited utility in the acute management of childhood asthma. A more definitive answer for the utility of feNO in the acute setting may be obtained in a study that follows the patients over a longer duration than the typical 2-4 hour period covered by Kwok et al. [56]. Although it may have been harder for children to manage the respiratory maneuvers for the feNO determination, it may be a more reliable tool for the adult asthmatic population in acute care settings. Finally, an easier to use device may be the answer. More recently, the portable NIOX-MINO, mentioned above, has shown a high correlation (r = 0.97, P < 0.001) with the more conventional chemiluminescence device (NIOX) [57]. More encouraging was that in the 55 children enrolled (4-9 years of age), up to 73% successfully provided feNO measurements, and thus this may be a better tool for screening as well as management of acute asthma in the pediatric and adult population [57].
Since NO is a ubiquitous signaling molecule, perturbations in the NO pathway in the asthmatic airway over time may be reflected in changes in levels of NO metabolites measured elsewhere, such as plasma and urine. Determining ratios between the levels of NO and its metabolites by sampling different compartments may reveal a time course reflecting evolution of the disease. Thus we may be able to monitor NO from different compartments in different settings (first in the ED and then during critical care) and at different times indicating its biomarker potential for asthma severity and management. Having better tools to diagnose, predict and manage an acute asthma exacerbation would help reduce the burden of this illness, particularly in the pediatric population that is disproportionately affected by this problem.
NO and its Metabolites in Acute Kidney Injury
Acute kidney injury (AKI) is a common complication among ambulatory and hospitalized patients, and can cause significant morbidity and mortality, specifically in patients in critical care medicine [58-61]. Patients, including pediatric ones, who are or will get critically ill from AKI are likely to pass through the ED [3, 4]. As alluded to in the introduction to this review, the conventional biomarker for AKI, namely serum creatinine, can take up to 3 days for an elevation or change from baseline, indicative of worsening renal function [60, 62, 63]. Thus, additional early biomarkers are needed that can be diagnostic and prognostic of AKI in the ED and critical care settings, at a time when baseline serum creatinine is either unavailable or uninformative. NO has been shown to have several functions in the kidney, depending on its concentration, site of release, and duration of action [64]. Endothelial injury and the subsequent microcirculatory dysfunction is likely a primary event in renal hypoxia with disturbances in NO-related pathways playing key roles [65]. In an adult post-ischemic model of AKI urinary concentration of NO (as measured by its metabolites nitrate and nitrite) were markedly lower suggesting that this was a reflection of potential endothelial damage in the kidney [66]. Thus, we conducted a study to test the hypothesis that urinary concentration of nitrate and nitrite would be decreased in early AKI in children presenting to the ED [4]. We showed that in 252 patients, 0-19 years of age presenting to the pediatric ED, lower concentration of urinary nitrate, but not nitrite, was significantly associated with AKI (Fig. 3), thus indicating the potential for this as a novel urinary biomarker for AKI in the ED [4]. More encouraging was the potential for this urinary biomarker in the face of a normal serum creatinine in almost half of those with AKI [4]. Future studies are needed to evaluate fully the ability of urinary nitrate to predict important in-patient clinical outcomes, such as critical care needs, dialysis, and ultimately mortality. As mentioned above, urinary NO metabolites are a reflection of both dietary intake of NOx as well as endogenous generation of NO, thus additional studies are needed to determine whether diminished level of urinary nitrate in AKI is truly indicative of renal endothelial dysfunction.
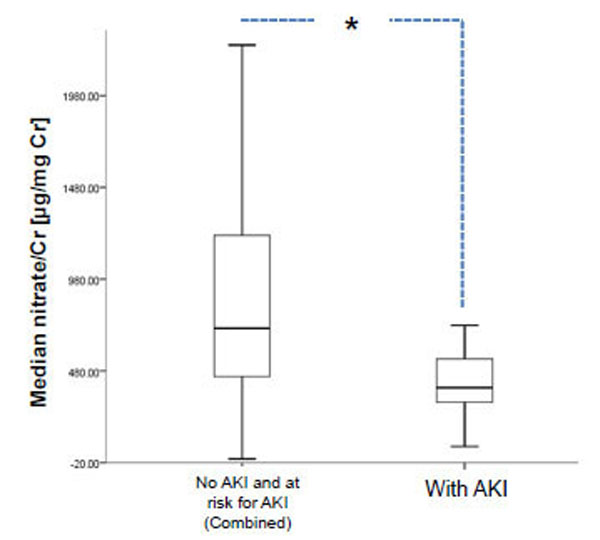
Urinary nitrate distinguishes patients with acute kidney injury (AKI) from those either at risk or without AKI. Data based on ref. [4], shows median urinary nitrate normalized to urinary creatinine in patients presenting to TCH [N=252]. Bar on left represents combined no AKI and at risk for AKI, whereas the one on the right represents those with AKI. Data are shown as box plots with the heavy line in the box representing the median (50th percentile) and the ends of the box representing 25th and 75th percentiles, respectively. Groups were compared using the non-parametric Mann Whitney U test (*P=0.02). Cr, creatinine. TCH, Texas Children’s Hospital.
NO and its Metabolites in Sepsis and Septic Shock
Sepsis is defined as the systemic response to infection, and about half of those patients progress to septic shock characterized by refractory hypotension and multiple organ failure [6]. Severe sepsis and septic shock are associated with significant mortality, as high as 28% [67]. Hemodynamically, both macro- as well as microcirculatory failure is the hallmark of severe sepsis and septic shock [67]. The central role of NO in maintaining microcirculation is highlighted in sepsis and septic shock, states in which the microcirculation has sustained an insult. Several studies have implicated the overproduction of NO as the cause for the decompensated state [6]. Sepsis, septic shock or systemic inflammatory response syndrome in intensive care settings is associated with increased plasma nitrate and nitrite [68-70]. Measuring both plasma and urinary NOx has revealed elevations in patients with sepsis and septic shock [6]. Innate immune pathway activation in response to infection and inflammation, particularly in sepsis and shock, is associated with increased activity of iNOS, and thus more NO being released [20, 71]. However, studies in experimental animals reveal that iNOS upregulation and production of NO may have a feedback inhibition on constitutive NO production in other tissues [21, 72]. Urinary NOx reflects both the production and utilization of NO [73, 74]. Nitrate is the main urinary NO metabolite, whereas nitrite is low to undetectable in urine [75]. Sepsis and shock can expose the kidney to ischemic injury and this can be reflected in low levels of uNOx excretion likely due to low endogenous NO generation [4, 66]. Conversely, since the majority (96%) of filtered nitrate and nitrite is reabsorbed by the renal tubules, high levels in urine secondary to reduced renal reabsorption may reflect acute renal failure concomitant with sepsis/septic shock [4, 76].
Although NO directly as well as by conversion to peroxynitrite can be bactericidal, excessive levels are counterproductive as evidenced by the refractory vasodilation, hypotension and decreased tissue perfusion [6]. Irrespective of mechanism and level of metabolite, NO as a downstream effector of innate immune activation lends itself as a potential biomarker for sepsis and septic shock. This may be particularly useful in the acute setting as the concentration of NOx in plasma or urine may predict important in-patient clinical outcomes, such as need for hospitalization, level of support (intensive care versus regular floor), and mortality.
NO and its Metabolites in Acute Myocardial Infarction: Going Beyond Diagnostic Biomarkers to Therapeutic Intervention
As stated at the outset a continuous endogenous supply of NO is crucial for the physiological integrity of the cardiovascular system. In fact, an imbalance in the production or bioavailability of NO is unequivocally linked to cardiovascular pathology. With more recent advances in establishing the nitrate-nitrite-NO pathway, the reverse pathway that enables more NO to be generated when endogenous NOS pathways are dysfunctional, harnessing it therapeutically in prevention and treatment of cardiovascular disease becomes self-evident [77-79]. Ischemic heart disease is a major player in the acute care setting. In this disease process where regional hypoxia and ischemia prevail, it may be of great benefit to replenish the stores of NOx either pharmacologically or through diet [77, 78]. In the past, dietary NOx had been granted pariah status because of nebulous links to disease [78]. With greater evidence suggesting benefits of the oxidative NO metabolites in ischemia/reperfusion injury, a real paradigm shift is needed in the scientific community from the perspective of the relatively simple inorganic anions nitrate and nitrite. Nitrite, in fact, may be the endocrine mediator of NO-based signaling [80]. Bolusing nitrite intravenously prior to instigating a cardiovascular insult has been shown to significantly reduce the injury not only from myocardial infarction, but also ischemic liver and kidney injury and stroke in multiple animal models [81-84]. These pre-clinical or benchwork-based experiments have prompted several human clinical trials on NOx for cardiovascular complications like acute ischemia. In a very promising study nitrite therapy was shown to improve cardiac and neurological function and overall survival post cardiac arrest [85]. Initiating NOx-based clinical efficacy trials in the acute setting are justified since that is where cardiovascular complications like acute MI and cardiac arrest are first encountered by the medical team. These interventions will likely improve acute care management from the cardiovascular morbidity and mortality perspective.
CONCLUSIONS AND FUTURE DIRECTIONS: ACUTE CARE AND BEYOND
This review has focused on the role of NO and its metabolites in a few disease processes commonly encountered in the acute care setting (sumarized in Fig. 2). We have argued that NO and its metabolites nitrate and nitrite can be potential biomarkers that need to be developed further from a diagnostic and prognostic perspective and that is likely to improve management in the acute care setting. Measured exhaled NO (feNO) concentration is immediately available and may be a reliable tool for assessment of airway inflammation in an acute asthma exacerbation. Concentration of NOx in multiple biological samples (plasma, urine, nasal secretion, CSF) measured via HPLC can also be reliably obtained in a rapid manner since the HPLC run per sample takes an average of 8 minutes [8]. Samples can be batched and run on the HPLC. Hence, both NO and NOx have the potential of being rapidly determined biomarkers for multiple disease processes encountered in the acute care setting (summarized in Fig. 2).
There are limitations to using NO and it’s metabolites as biomarkers. Although NOx can give a fairly reliable idea of systemic NO bioavailability, its concentration in urine and plasma being heavily dependent on renal activity, it cannot be an entirely accurate measure of NOS functionality. Additionally, the presence of nitrite and nitrate in everyday diet adds another restriction towards using NOx in plasma and urine as absolutely indicative of disease process [8]. Single feNO or NOx determinations simply may not be enough. Thus, sequential measurements of the biomarkers may be needed to get a better handle on the evolution of the disease process, initially as the patient presents in the acute phase and then during the course of critical care. Further, sampling from multiple compartments may be more informative of the disease process. For instance, for AKI, plasma and urinary NOx measurements simultaneously would enable us to determine the fractional excretion of urinary NO metabolites, potentially a more informative parameter for the disease entity than urinary NOx alone.
Given the non-specific nature of NO and its metabolites, they will be unlikely to entirely replace pre-existing tests. Hence, this type of analysis should be in addition to the standard diagnostics and biomarkers. Having a more comprehensive picture will only improve diagnosis, prognosis and effectiveness of treatment and reveal how NO is or may be playing a role in the acute disease process. NO-based analyses might not be able to differentiate between primary pulmonary versus cardiac disease. However, in the setting of a relevant clinical presentation and work up, analyzing NO and metabolites might be a valuable assessment for a NO component, and as such, an adjunct.
General state of health and co-morbid disease states might influence results, and hence have significant limitations on the use of NO or NO metabolites as biomarkers. Dietary NOx, as alluded to above, is a potentially significant limitation due to the fact that in the ED and critical care settings we cannot always predict or select patients based on fasted states or dietary patterns.
There is obvious value in obtaining a non-invasive specimen for determining levels of biomarkers for disease states. This will require the development, validation and then clinical usage of relatively simple, rapid and cost-effective techniques that may sample multiple body fluids collected non-invasively, such as nasal washes, saliva and urine [86]. Future studies will improve our understanding of NO pathophysiology and thus enable us to rationally devise pharmacological treatment for manipulating NO in acute disease states. While many of these therapeutic approaches remain speculative, better understanding of the underlying mechanisms that regulate NO function will ultimately improve our ability to implement such therapies. Adaptation to and validation of a point-of-care test with the assistance of an industry partner will enable inexpensive screening capacity on a dipstick, point-of-care type of a platform, to aid treatment decisions [87]. This has obvious global health implications that go far beyond any specific clinical setting [87].
Overall, what is most importantly highlighted, for acute care and non-acute care physicians alike, is the ongoing research need to determine the physiological roles of NOx, and if it can be used unequivocally both as diagnostic biomarker of disease and for treatment regimens, in a variety of clinical settings.
CONFLICT OF INTEREST
The authors confirm that this article content has no conflicts of interest.
ACKNOWLEDGEMENTS
None declared.
REFERENCES
[PubMed Link]
[PubMed Link]
[PubMed Link]
[PubMed Link]
[PubMed Link]
[PubMed Link]
[PubMed Link]
[PubMed Link] [PMC Link]
[PubMed Link]
[PubMed Link]
[PubMed Link] [PMC Link]
[PubMed Link]
[PubMed Link] [PMC Link]
[PubMed Link]
[PubMed Link]
[PubMed Link]
[PubMed Link]
[PubMed Link]
[PubMed Link]
[PubMed Link]
[PubMed Link]
[PubMed Link]
[PubMed Link]
[PubMed Link]
[PubMed Link]
[PubMed Link] [PMC Link]
[PubMed Link]
[PubMed Link] [PMC Link]
[PubMed Link] [PMC Link]
[PubMed Link]
[PubMed Link]
[PubMed Link]
[PubMed Link]
[PubMed Link]
[PubMed Link] [PMC Link]
[PubMed Link]
[PubMed Link]
[PubMed Link] [PMC Link]
[PubMed Link]
[PubMed Link]
[PubMed Link]
[PubMed Link]
[PubMed Link]
[PubMed Link]
[PubMed Link]
[PubMed Link]
[PubMed Link]
[PubMed Link] [PMC Link]
[PubMed Link] [PMC Link]
[PubMed Link]
[PubMed Link]
[PubMed Link]
[PubMed Link]
[PubMed Link]
[PubMed Link]
[PubMed Link] [PMC Link]
[PubMed Link] [PMC Link]
[PubMed Link]
[PubMed Link]
[PubMed Link]
[PubMed Link] [PMC Link]
[PubMed Link]
[PubMed Link]
[PubMed Link]
[PubMed Link]
[PubMed Link]
[PubMed Link]
[PubMed Link]
[PubMed Link]
[PubMed Link]
[PubMed Link]
[PubMed Link] [PMC Link]
[PubMed Link] [PMC Link]
[PubMed Link] [PMC Link]
[PubMed Link]
[PubMed Link]
[PubMed Link] [PMC Link]
[PubMed Link]
[PubMed Link]