All published articles of this journal are available on ScienceDirect.
The Involvement of Xanthohumol in the Expression of Annexin in Human Malignant Glioblastoma Cells
Abstract
Glioblastoma multiforme (GBM) is the most common malignant and resistant tumor of the central nervous system in humans and new therapeutic strategies are urgently required. Recently, we have shown that the potential chemotherapeutic polyphenol xanthohumol (XH), isolated from Humulus Lupulus, induces apoptosis of human T98G glioblastoma cells by increasing reactive oxygen species and activating MAPK pathways. Then we have found, by western blotting and microscopic analysis, that XH up-regulates cytosolic levels of ANXA1 and induces translocation of the protein on the cell membrane of T98G cells in a time-dependent manner with significant effects observed after 24 h. On the basis of the above evidence, the aim of this work was to investigate the role of intracellular and cell membrane localized ANXA1 in GBM cells. RT-PCR analysis has shown that XH up-regulates mRNA levels of ANXA1 after 16 h treatment. To demonstrate the involvement of ANXA1 in apoptosis of GBM cells we down-regulated ANXA1 expression with small interfering RNA (siRNA) and then analysed apoptosis in the presence and absence of apoptotic stimuli. Importantly, apoptosis induced by XH was reduced in siRNA-ANXA1 transfected cells where western blot analysis shows a significant reduction of ANXA1 protein levels. To investigate the role of ANXA1 expression on the cell membrane of T98G cells as potential “eat-me” signal we studied phagocytosis of apoptotic cells by human macrophages. We incubated apoptotic T98G cells with human blood monocyte derived macrophages (M=). After co-incubation period we analysed the percentage of M= phagocytosing the apoptotic cells by cytofluorimetric FACS analysis and by confocal microscopy. Our results show that XH induces phagocytosis of apoptotic T98G cells by human M= in a concentration-effect manner, a processes that is dependent on caspase mediated apoptosis. ANXA1 acts as an “eat-me” signal on the cell membrane of T98G cells, and interestingly, apoptotic siRNA-ANXA1 transfected cells are not completely ingested by M=. These results were confirmed by incubating apoptotic cells with a neutralizing anti-ANXA1 antiboby and ANXA1 membrane depletion by EDTA washing. ANXA1 was also detected in supernatants of apoptotic cells and the incubation of enriched supernatants enhanced the percentage of phagocytosis by M=. These results demonstrated that ANXA1 is involved both in the apoptosis and phagocytosis of glioblastoma cells. This study shows a possible role of ANXA1 in maintenance of brain homeostasis and may lead to novel therapeutic approaches for neuro-inflammatory diseases and chemotherapy targets in the treatment of glioblastoma multiforme.
INTRODUCTION
GBM (WHO grade IV) is the most common tumor of the central nervous system (CNS) in humans and is particularly highly aggressive and invasive exhibiting rapid cell growth, resistance to apoptosis and robust angiogenesis. Unfortunately, the median survival of GMB patients is 6-12 months from the time of diagnosis and current treatments, including surgery, radiation therapy and chemotherapy have not significantly improved the prognosis [1]. The major limitations of chemotherapy for the treatment of GBM are the inability of many drugs to pass through the blood-brain barrier and their low efficacy to induce apoptosis. Therefore the choice of effective drugs is limited and new therapeutic strategies urgently need to be explored. There is increasing evidence that several natural compounds found in plants may be useful as cancer chemopreventive or chemotherapeutic agents. Xanthohumol (XH) is one of the major prenylated phenolics occurring in the hop cones of Humulus lupulus L.. Hops are traditionally used to add bitterness and flavor to beer, however, more recently alternative uses for hop polyphenols and their effect on several biological processes have become apparent. Limited in vitro studies indicate that several prenylated flavonoids present in the hop plant possess anticancer properties. In particular, XH has received major attention because it has been shown to inhibit initiation, promotion and progression stages of carcinogenesis, hence appearing as a broad-spectrum chemopreventive agent [2]. Anticancer activities of several beer polyphenols, including XH, have been investigated in various cell lines. For example, XH inhibits human breast (MCF-7), colon (HT-29), ovarian (A2780), prostate (DU145, PC-3) cancer [3] and B-chronic lymphocytic leukemia cell proliferation [4] and induces apoptosis in human breast [5] and prostate cancer cells in vitro [6]. In addition, pro-apoptotic effects of a hop-bitter acid, humulone (H), in the leukemia cell lines, HL60 and U937, have also been reported [7]. A recent in vivo study showed the ability of xanthohumol to induce a significant inhibition of angiogenesis in mice implanted with a matrigel sponge when administered in the drinking water at a concentration of 2 µM. At higher concentration of 200 µM XH displayed a marked angiogenesis inhibition without adverse effect on animal health parameters [8]. It has also been shown that XH targets tumor growth and angiogenesis in Kaposi’s sarcoma tumor in male nude mice [9].
ROS, such as superoxide anion (O2.-), hydrogen peroxide (H2O2) and hydroxyl radical (HO.) influence many biological processes, including apoptosis [10]. The redox state of the cells is a crucial factor in deciding its susceptibility to apoptotic stimuli [11]. ROS at low concentrations play a role as intracellular messengers regulating several processes including cell proliferation, while the production of large amounts of ROS promote apoptosis [12]. Flavonoids are generally considered to be antioxidants, but most of them behave as powerful pro-oxidant molecules [13-15]. XH is structurally related to flavonoids and although may exert antioxidant activity, it also appears to increase the cellular content of ROS [16]. It has been found that XH-induced apoptosis in leukemic K562 cells was associated with elevation of cellular ROS content and that the antioxidant N-acetylcysteine (NAC) blunted XH induced apoptosis [17].
Flavonoids can also trigger apoptosis through modulation of different signal transduction pathways, such as the phosphatidyinositol-3-kinase/Akt and mitogen activated protein kinase (MAPK) pathways, which may affect cellular function by modulating genes regulation or phosphorylating proteins [18]. Furthermore, they can directly or indirectly affect the activity and expression of key proteins (e.g., caspases, Bcl-2 family members) involved in the regulation of apoptosis, It has been found that XH-induced apoptosis in K562 cells was associated with a prolonged stimulation of ERK phosphorylation [19]. Moreover, recently it has been reported that certain flavonoids induce apoptosis in human glioblastoma T98G and U87MG cells, but not in human normal astrocytes through increase of ROS production, changes in mitochondria membrane potential (Δψm) and phosphorylation of MAPKs [20].
Recently, we have shown that the potential chemotherapeutic polyphenol xanthohumol (XH), isolated from Humulus Lupulus, induces apoptosis of human T98G glioblastoma cells by increasing reactive oxygen species and activating MAPK pathways [21]. Then we have found, by western blotting and microscopic analysis, that XH up-regulates cytosolic levels of ANXA1 and induces translocation of the protein on the cell membrane of T98G cells in a time-dependent manner with significant effects observed after 24 h. On the basis of the above evidence, the aim of this work was to investigate the role of intracellular and cell membrane localized ANXA1 in GBM cells. RT-PCR analysis has shown that XH up-regulates mRNA levels of ANXA1 after 16 h treatment. To demonstrate the involvement of ANXA1 in apoptosis of GBM cells we down-regulated ANXA1 expression with small interfering RNA (siRNA) and then analysed apoptosis in the presence and absence of apoptotic stimuli.
MATERIALS AND METHODS
Cell Cultures
Human glioblastoma T98G (WHO grade IV) and U87-MG (WHO grade III) cell lines, obtained from ATCC, were cultured in DMEM medium supplemented with 2 mM L-glutamine, 10% heat-inactivated fetal bovine serum (FBS), 1% sodium pyruvate, 1% non-essential amino acids and 1% penicillin/streptomycin (all from Lonza, Switzerland) at 37°C in 5% CO2 humidified incubator. The cells were used up to a maximum of 10 passages.
Western Blot Analysis
Expression of ANXA1 on cell surface and cytosolic level was evaluated by Western blot analysis. For cell surface proteins, cells, kept on ice, were washed with PBS containing protease and phosphatase inhibitors (1mM PMSF, 10 µg/ml leupeptin, 10 µg/ml pepstatin, 10 µg/ml aprotinin, 1 mM Na3VO4, 1 mM NaF; Sigma-Aldrich, Milan, Italy) and 5 mM EDTA (Sigma-Aldrich, Milan, Italy), which acts as a Ca2+ chelating agent, removing the ANXA1 bound to the cell membrane. The intracellular proteins were extracted from cells by a lysis buffer (50 mM Tris-HCl, 1% Na-deoxycholate, 1% SDS and 0.5% IGEPAL) containing protease and phosphatase inhibitors (1mM PMSF, 10 µg/ml leupeptin, 10 µg/ml pepstatin, 10 µg/ml aprotinin, 1 mM Na3VO4, 1 mM NaF; all supplied by Sigma-Aldrich, Milan, Italy).
The lysed cells were then centrifuged at 12,000 xg at 4°C for 10 min. The supernatant is the extract of proteins. Protein content was estimated according to Biorad protein assay (BIO-RAD, Milan, Italy). Protein samples (30 µg) were loaded onto 10% acrylamide gel and separated by SDS-PAGE. The separated proteins were then transferred electrophoretically (100 mA Blot for 90 minutes; Trans Blot semidry, Biorad) to nitrocellulose membrane (Immobilon-NC, Millipore, Bedford, USA), using a transfer buffer [25mM Tris, 192 mM glycine (Sigma-Aldrich, Milan, Italy) and 20% v/v methanol (Carlo Erba, Milan, Italy)]. The non-specific binding were blocked by incubation of membrane in TBS-TWEEN 0.1% (25 mM Tris; 150 mM NaCl, 0.1% v/v Tween) with 5% nonfat dry milk (BIO-RAD, Milan, Italy) in for 60 minutes. Subsequently, the membranes were incubated overnight at 4°C with primary antibodies: anti-rabbit polyclonal ANXA1 (Invitrogen) and anti-β-actin monoclonal 93 mouse (Sigma-Aldrich, Italy). After incubation with primary antibodies and 3 washes in TBS-Tween 0.1%, were added specific secondary anti-rabbit or anti-mouse, diluted 1:5000, (both supplied by Sigma-Aldrich, Milan, Italy) for 1 hour at room temperature. The specific signals were visualized by chemiluminescence detection system (GE Healthcare, Milan, Italy). Digital images were obtained on a ImageDoc 2000 equipment (BIO-RAD, Milan, Italy).
Analysis of Apoptosis
Hypodiploid DNA was analysed using the method of propidium iodide (PI) staining and flow cytometry as described by Riccardi and Nicoletti (2006). Briefly, cells were washed in phosphate-buffered saline (PBS) and resuspended in 500 µl of a solution containing 0.1% sodium citrate, 0.1% Triton X-100 and 50 µg/ml propidium iodide (Sigma-Aldrich, Milan, Italy). After incubation at 4C° for 30 minutes in the dark, cell nuclei were analyzed with Becton Dickinson FACScan flow cytometer using the Cells Quest program. Cellular debris was excluded from analysis by raising the forward scatter threshold, and the DNA content of the nuclei was registered on logarithmic scale. The percentage of the cells in the hypodiploid region was calculated.
Transfection with Small-interference RNA (siRNA) Anti-ANXA1
Two small interference RNAs (siRNA) were designed for ANXA1: ANXA1 (A) (sense 5'-CAGCGUCAACAGAUCAAAG-3') and ANXA1 (B) (sense 5'-CCGAUCUGAGGACUUUGGU-3' (Dharmacon Research Inc., Lafayette, CO, USA). The scrambled siRNA oligo-KROAA OO6461 (sense 5'-CAGUCGCGUUUGCGACUGG-3') (Dharmacon Research Inc., Lafayette, CO, USA) was used as control. 1.5x105 cells were plated in 6-well multiwell plate reaching a confluence of 30-50% and kept in DMEM containing 10% FBS. The transfection was performed using oligofectamine (Invitrogen, Milan, Italy) according to manufacturer’s instructions, and obtaining a final mixture of RNA (A) and (B) 100nM. After 48 hours, the cells are treated in the presence and in the absence of XH (20 μM) and analyzed by flow cytometry and western blot.
Real-time RT-PCR Analysis
Total RNA was isolated from T98G cells using TRIzol Reagent (Invitrogen, Carlsbad, CA, USA) according to manufacturer’s instructions. The RNA quality was assessed by agarose gel electrophoresis. The RNA quantity was measured using a spectrophotometer. Three micrograms of isolated RNA were reverse-transcribed using oligo(dT) primer and SuperScriptII (Invitrogen, Carlsbad, CA, USA) in a total volume of 20 μl. Real-time PCR was performed with Light-Cycler® System Ver. 3.5 (Roche Diagnostics GmbH, Mannheim, Germany) using SYBR Green detection in a total volume of 20 µl with 1 µl of forward and reverse primers (10 mM) and 10 µl of SYBR GreenPCRMaster-Mix (Roche Diagnostics GmbH, Mannheim, Germany). Reactions included an initial cycle at 95°C for 10 min, followed by 40 cycles of denaturation at 95°C for 10 sec, annealing at 55°C for 5 sec, extension at 72°C for 15 sec. Values were determined from standard curve generated from serial cDNA dilutions and normalized to GAPDH. Fold change of induction were determined by calculating ratios between control and treatment normalized signals. Differences between mean values were evaluated for statistical significance using the unpaired Student t-test. (Differences were considered significant with P<0.05.) Primers used for real-time PCR are as follows: ANXA1 forward 5’-ATCAGCGGTGAGCCCCTATC -3’ and reverse 5’- TTCATCCAGGGGCTTTCCTG -3’, GAPDH forward 5’- AATTCCATGGCACCGTCAAGG -3’ and reverse 5’- TCGCCCCACTTGATTTTGGAG -3’.
CONFOCAL MICROSCOPY
T98G cells were seeded on 12mm slides with their culture medium and after 24 hours were treated with xanthohumol 20 µM for 24 hours. After incubation time the culture medium was removed and cells in adhesion on glass slides were washed twice with PBS. Subsequently, the cells were fixed with para-formaldehyde at 3.7% for 20 minutes and then washed with PBS. Then it was added a 0.1M glycine solution and incubated for 5 minutes. After washing with PBS, the cells were incubated with 1% BSA in PBS for 1 hour. The cells were then incubated with the primary antibody polyclonal anti-ANXA1 (1:100) (Zymed, Invitrogen) for 2 hours. Cells were also incubated with DAPI (4,6-diamidin-2-phenylindole) (1:1000) to obtain a staining of cell nuclei. Subsequently the cells were washed 2 times with PBS and incubated with the secondary antibody Alexa Fluor 647 anti-rabbit for 30 minutes. After 2 more washes with PBS the slides were mounted on the door slides with Moviol (3-4μl/vetrino). The slides were kept in cells down until the reading performed with Leica confocal microscope and observed with a 63X magnification.
Activity Assay of Caspase-3
The activity of caspase-3 in cellular extracts was determined analyzing the release of 7-amino-4-methylcoumarin (AMC) from N-acetyl-DEVD-AMC (Nicholson, 1995). The cells (2x106) were lysed in a buffer containing 10 mM Tris-HCl, 10 mM NaH2PO4/NaHPO4 (pH 7.5), 130 mM NaCl, 1% Triton -X-100, 10 mM NaPPi (sodium pyrophosphate). 20μg of protein were incubated with 20 μM Ac-DEVD-AMC (Becton Dickson) in the reaction buffer [ 20 mM HEPES (pH 7.5), 10% glycerol and 2 mM dithiothreitol (DTT)] at 37°C for 2 hours. AMC release is monitored in a spectrofluorometer at an excitation wavelength of 380 nm and an emission wavelength range of 430-460 nm (L55 Luminescence Spectometrer, Perkin Elmer Instruments).
STATISTICAL ANALYSIS
All results are mean ± SEM of 3 experiments performed in triplicate. The optical density of the protein bands detected by Western blotting was normalized on β-actin levels. Statistical comparison between groups were made using Bonferroni parametric test. Differences were considered significant if p < 0.05.
RESULTS
Effects of Xanthohumol on the Induction of Apoptosis in Different Cell Lines of Glioblastoma
In the first phase of this work, we evaluated the apoptosis induced by xanthohumol in different glioblastoma cell lines with different degrees of malignancy the T98G (WHO IV), the U87-MG (WHO III-IV) and U343-MG (WHO III). It was therefore verified the effect on apoptosis of xanthohumol, at various concentrations and at different times, in different lines of glioblastoma with a different degree of malignancy. The cells were incubated for 8, 24 and 48 hours in the presence and in the absence of xanthohumol to different concentrations 10, 20, 50 μM. At the end of the incubation periods of all three cell lines were harvested to assess apoptosis by measuring the percent of hypodiploid cells by flow cytometry. The data reported in Fig. (1A) show that the effects of xanthohumol induces apoptosis in T98G cells already at 24 hours with an effect massimoa 48 hours after treatment at a concentration of 20 uM. Fig. (1B and C) shows the effects of xanthohumol in different concentrations and at different times on the other two cell lines, the U-87 and U-343 with a lower degree of malignancy. Xanthohumol is capable of inducing a small effect on apoptosis of U87MG cells only at the highest concentration at 48 h (p <0.05 vs. CTR) while in U343MG cells, xanthohumol gives no significant effect to the times and at the concentrations used. These results revealed that the T98G cells are much more sensitive U87-MG and U343-MG to the action of xanthohumol. Based on this result we have chosen the use of XH 20 uM concentration as the optimal treatment conditions in T98G cells of glioblastoma multiforme.
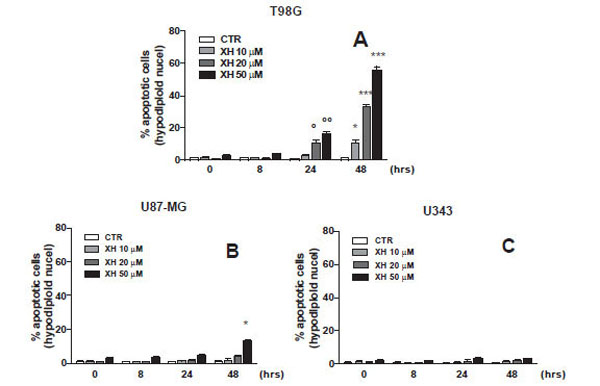
Dose/effect curve at different administration time of three different cell lines of glioblastoma (A) T98G, (B) U87-MG and (C) U343-MG. Apoptosis was induced by xanthohumol at different concentrations (10-20-50µM) after administration at different times (8-24-48 hours) and measured by flow cytometry, as a percentage of hypodiploid nuclei by permeabilization and incorporation of iodide of propridio (PI). Data are shown as mean ± SEM of three experiments performed in triplicate. (A) ° P <0.05, ° ° P <0.01 vs CTR T98G cells 24 h, * P <0.05, ** P <0.01, *** P <0.001 vs CTR T98G cells 48h. (B) * P <0.05 vs. CTR cells U87-MG 48 h.
Effects of the Xanthohumol on the Total Expression of ANXA1 in Various Cell Lines of Glioblastoma
To verify a possible modulation of the expression of ANXA1 by xanthohumol, the three different cell lines of glioblastoma (T98G, U87-MG and U343-MG) were incubated in the presence or absence of xanthohumol (20μM) at the times indicated in graph. After obtaining the total cellular protein extracts, 35μg of protein were analyzed by Western blotting using polyclonal anti-ANXA1. The immunoreactive protein bands were detected by chemiluminescence and quantized by densitometric analysis, normalizing the levels of the protein on the expression of actin obtained in the same extracts. Fig. (2) shows that the effect of xanthohumol on the modulation of the expression of ANXA1 is found only in T98G cells, in fact there is no change in the levels of expression of ANXA1 nor in U87-MG cells nor in U343-MG cells. Fig. (2) shows that the effect of xanthohumol in T98G on the expression of ANXA1 is time-dependent. Xanthohumol induces a significant increase in protein levels at 16 hours after stimulation, with a maximum peak of expression at 48 hours.
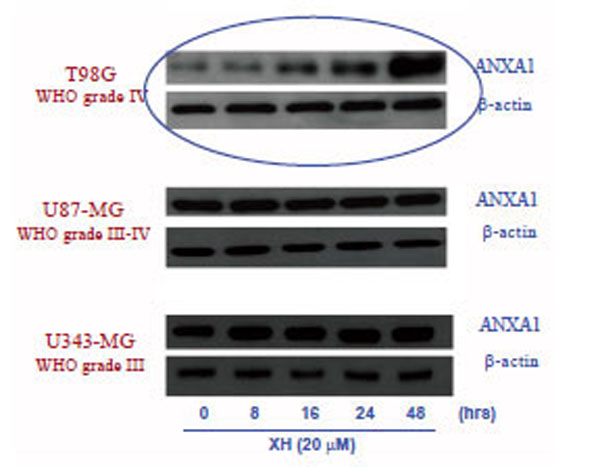
Effects of xanthohumol on the expression of ANXA1 in three different glioblastoma T986, U87-MG and 4343-MG.cell lines: The three cell lines were incubated in the presence and in the absence of xanthohumol 20µM at different time points (0-48 h). The expression of ANXA1 was analyzed by Western blotting, using a polyclonal anti-ANXA1 and normalizing the amount of protein analyzed by an antibody anti-β-actin.
Effects of Xanthohumol on the Expression of ANXA1 in the Cytosol and Plasma Membrane in T98G Cells
On the basis of interesting preliminary data obtained about apoptosis and the expression of ANXA1 following treatment with xanthohumol, in different cell lines of glioblastoma, we decided to study the role of ANXA1 in apoptosis induced by XH in cells of glioblastoma multiforme T98G where the expression of protein by the XH was positively regulated. So we have assessed the possible modulation of expression of ANXA1 by xanthohumol in the cytosol and on the membrane of T98G cells. The cells were plated at a density of 5x106 cells / ml and incubated in the presence and in the absence of xanthohumol (20 μM) at the times indicated by Fig. (3A). After obtaining the extracts cytosolic, 30 μg of protein were analyzed by Western blotting using a polyclonal antibody directed against ANXA1. The immunoreactive protein bands were highlighted by means of chemiluminescence. The Western, in Fig. (3A) shows that the effect of xanthohumol on the expression of cytosolic ANXA1 is time-dependent. Xanthohumol induces a significant increase of the protein, with a peak expression at 48 hours. For the extraction of membrane proteins after the treatment times, the cells were incubated on ice with a solution of 5 mM EDTA PBS that acts as a chelating agent of Ca2+ and removes the ANXA1 anchored to the cell membrane via the Ca2+. Subsequently 10 µg for membrane proteins were analyzed by Western blotting, using a polyclonal anti-ANXA1. The Western, in Fig. (3B), shows that the expression of ANXA1 on the membrane surface increases already at 16 hours after stimulation with a peak expression at 48 hours. Xanthohumol is capable of promoting the translocation and thus the exposure of the protein on the membrane of T98G cells. To confirm the data obtained by Western blotting, the expression of ANXA1 on the membrane, following treatment with 20μM XH, has been evaluated through fluorescence microscopy performed with a confocal microscope (Leica) using a 63X magnification. The cells were plated on 12 mm slides and kept in culture for 24 hours before performing the treatments with XH. At the end of the incubation period the cells were washed and incubated with the primary antibody and polyclonal anti-ANXA1 contemporanemanete were also incubated with DAPI (4,6-diamidin-2-phenylindole) to obtain a staining of cell nuclei. The red color in the image of Fig. (3C) shows an accumulation of ANXA1 on the cell membrane as a result of treatment with xanthohumol to 24 hours thus confirming the data obtained previously by western blotting.
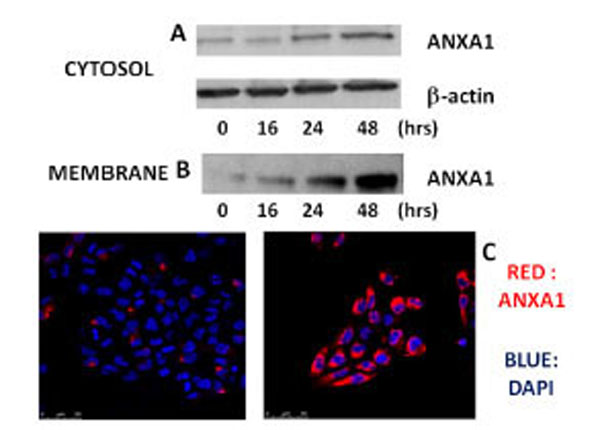
Effects of xanthohumol on the expression of ANXA1 in the cytosol and on the membrane of human T98G glioblastoma multiforme cells. (A) Analysis of the expression of ANXA1 in the cytosol of T98G cells. (B) Expression of levels of ANXA1 on the plasma membrane of cells T98G. T98G cells were incubated in the presence or absence of xanthohumol (20µM) at different times. The expression of ANXA1 was analyzed by Western blotting using a polyclonal anti-ANXA1. (C) Expression of levels of ANXA1 on the plasma membrane of T98G cells analyzed by confocal fluorescence microscopy. After 24 hours of incubation the cells were treated with 20µM xanthohumol for the stated time, subsequently the cells were washed and incubated with a polyclonal antibody anti-ANXA1 and with DAPI to have a colored of the nuclei. The intense red color that is seen in the figure is indicative of the presence of ANXA1 on the membrane.
Effects of Xanthohumol on the Transcriptional Regulation of ANXA1
Based on the results obtained previously, we wanted to investigate the effects of xanthohumol at the transcriptional level thus assessing the levels of mRNA for ANXA1. After treating the cells with 20μM xanthohumol at different times (8, 16, 24), we performed a real-time PCR. The amplifications and RT-PCR analysis were performed on a Light-cycler system (Roche) using the kit Light Cycler Fast Start DNA Master plus SYBR Green I (Roche Molecular Biochemicals, Mannheim, Germany). ANXA1 and the GADPH gene were amplified with specific primers and then the mRNA levels of ANXA1 were normalized with those of GADPH. The analysis results show, as seen in Fig. (4), which XH induces an increase in mRNA levels for ANXA1 in a time-dependent manner with a peak at 24 hours thus confirming a transcriptional regulation of the protein by xanthohumol.
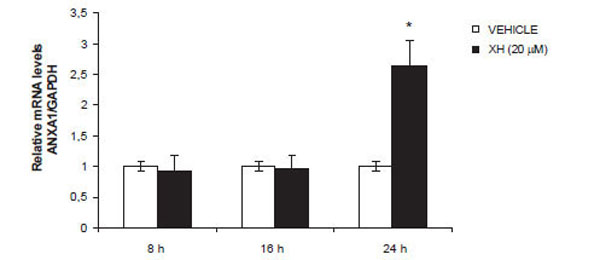
Effect of xanthohumol on ANXA1 mRNA expression. Fold change of induction were determined by calculating ratio between control and treatment normalized signals. Data are means ± SD of three independent experiments. * P≤0.01.
Effects of siRNA Directed Against ANXA1 on the Expression of ANXA1 in T98G Cells in the Presence and Absence of Xanthohumol
To evaluate the involvement of xanthohumol in the up-regulation of ANXA1 mRNA levels in T98G cells, we used small interference RNA directed against ANXA1 to down-regulate its expression. T98G cells, plated in multi-well 6-well, were transfected in the presence of oligofectamina containing a mixture of two siRNA directed against ANXA1 and simultaneously with siRNA-Scrambled used as a control. The cells were incubated with siRNAs for 48 hours and subsequently treated with xanthohumol (20μM). The protein extracts (30μg) were analyzed by means of Western blotting using a polyclonal anti-ANXA1 and an anti - actin for standardization. In control cells xanthohumol significantly increases the expression of ANXA1. The small interference directed against ANXA1 significantly inhibit the expression of ANXA1 both in the presence and absence of xanthohumol. The cells with siRNAs-scrambled present ANXA1 levels comparable to those of the control cells as evidenced by densitometric analysis shown in the graph (Fig. 5). The siRNAs directed against ANXA1 are able to significantly inhibit the expression of the protein both in the presence and absence of xanthohumol, in T98G cells.
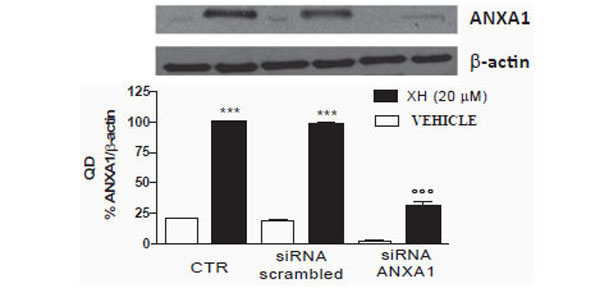
Effects of siRNAs directed against ANXA1 expression da togliere in T98G cells. T98G cells were transfected with oligofectamina containing concentrations of 100nM of small interference oligonucleotides directed against ANXA1 and scrambled control. After 48 hours of transfection, the cells were treated with xanthohumol 20µ for 24 h. Subsequently 30μg of protein were analyzed by Western blotting using a polyclonal antibody anti ANXA1. Data are shown as optical density, mean ± SEM of three representative experiments. Bonferroni test (*** P <0.001 vs CTR, ° ° ° P <0.001 vs scrambled xanthohumol and xanthohumol +).
Effects of siRNA Directed Against ANXA1 Apoptosis Induced by Xanthohumol in T98G Cells
To further analyze the effects of small interference directed against ANXA1 on apoptosis induced by xanthohumol, T98G cells were cultured with or without siRNA or siRNA-Scrambled-ANXA1. After 48 hours of transfection, the cells were treated with or without XH (20μM) and harvested after 48 hours to evaluate the percent of hypodiploid nuclei by flow cytometry. The data obtained show that, in cells transfected with scrambled siRNA induced apoptosis xanthohumol is almost unchanged compared to the cells not transfected and treated with xanthohumol. However in T98G cells transfected with siRNAs directed against ANXA1 apoptosis induced by xanthohumol is partially, but significantly inhibited (Fig. 6A). Then we also evaluated the activity of caspase-3 after transfection with siRNA-ANXA1 in T98G cells. The cells were transfected with and without siRNA-ANXA1 and siRNA-Scrambled and after 48 hours of incubation were treated with xanthohumol (20μM) for 8, 24 and 48 hours. After the incubation period with xanthohumol cells were used to measure the activity of caspase-3 by fluorimetric assay according to the method reported in the section materials and methods. The results obtained in Fig. (6B) show that the activity of caspase-3 has decreased significantly in cells transfected with siRNA-ANXA1 after 24-48 hours from treatment with xanthohumol compared to treatment with xanthohumol in cells not transfected with and xanthohumol in cells transfected with siRNA-Scrambled control. These data demonstrate that in the absence of ANXA1, the apoptosis induced by xanthohumol is significantly reduced confirming the involvement del'ANXA1 in the activation of caspase-3 and in the mechanism of cell death induced by xanthohumol.
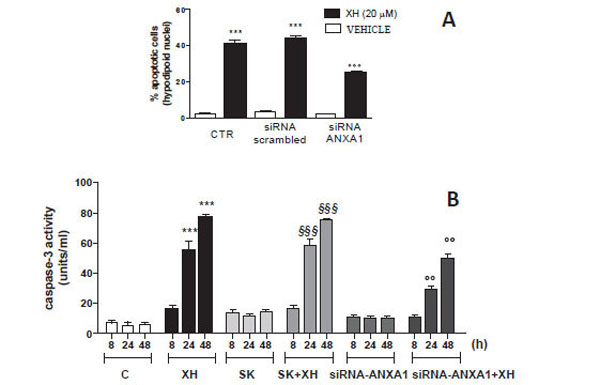
(A) Effect of siRNA-ANXA1 on apoptosis of T98G cells analyzed by staining with propidium iodide (PI) as an expression of hypodiploid nuclei. The cells were transfected for 48 hours with siRNA-ANXA1 (100nM) and siRNA-scrambled (SK) control and subsequently treated with xanthohumol for 24 hours. (B) Effect of siRNA-ANXA1 activation of caspase-3 in T98G cells measured by fluorimetric assay. The cells were transfected for 48 hours with si-RNA-ANXA1 (100nM) and subsequently treated with xanthohumol for 8-24-48 hours and then analyzed by fluorometric assay specific for caspase-3. Data are shown as mean ± SEM of three representative experiments representative. Bonferroni test (*** P <0.001 vs. control cells; § § § P <0.001 vs. control cells and cells transfected with SK, ° ° P <0.01 vs. cells treated with XH and XH + SK).
DISCUSSION
Glioblastoma multiforme is one of the most common cancers and malignant brain. The median survival for patients with GBM is 12.6 months from diagnosis for patients treated surgically and those treated with radiotherapy or chemotherapy. The prognosis of GBM is very poor, relative survival for adult patients diagnosed with GBM is 25% at one year, 5% in three years, 3% at five years, with no difference between men and women. The treatment of choice feasible in the case of GBM is definitely surgery and removal should be as radical as possible, even if in reality it is a highly invasive tumor complete resection is impossible and relapse is almost inevitable. Surgical therapy along with that radiotherapy and chemotherapy is part of palliative therapy that, though with modest results, allows to increase the duration of life of the patient. The drug most widely used for the treatment of GBM is temozolomide which is often used in combination with other chemotherapeutic agents to prevent the progression of the tumor. It is based on the lack of effective chemotherapeutic agents for the treatment of this tumor that arises the need always to search for new therapeutic valid strategies. In recent years, the natural products have been the focus of numerous studies, in order to find agents with potent antitumor activity. Some phenolic constituents extracted from hop cones have been shown to have a potential action for the prevention and treatment of various cancers.
Among the various components of the hops, xanthohumol, a chalcone prenilato of Humulus lupulus L., it was found a good chemopreventive and chemotherapeutic agent in many experimental models, both in vitro and in vivo, and has been shown to have a pro-apoptotic in T98G glioblastoma multiforme cells. In fact in a previous study, conducted in our laboratory, it has been demonstrated that apoptosis induced by XH is associated with the activation of caspase-3, caspase-9 and the cleavage of PARP, and is mediated by signaling pathway intrinsic mitochondrial and amplified by depolarization of the mitochondrial membrane, by the release of cytochrome c from the negative regulation of anti-apoptotic protein Bcl-2. It was also demonstrated that XH induces the release of intracellular ROS. The intracellar production of ROS is essential for the activation of the signaling pathway mitochondrial and the induction of apoptosis after exposure to XH. Oxidative stress caused by the treatment with the XH was also associated with activation of MAPK, as shown by the increase in the phosphorylation of ERK1 / 2 and p38. Xanthohumol is not able to induce cell death in primary cultures of cells astroticiche not transformant thus indicating a selectivity of action towards cancer cells of glioblastoma multiforme.
The ANXA1, initially characterized as a cytosolic protein with anti-inflammatory activity induced by glucocorticoids, over the past few years has shown to be present also at the level of the membrane and to be involved in various biological mechanisms phones as well as pathological, such as cancer. Of great interest in recent times is the study of the biological activities of ANXA1 including the inflammatory process, cell proliferation, the adjustment of the signals of cell death, apoptosis, and as demonstrated for the first time from an article by McKanna [22] , the phagocytic removal of apoptotic cells. It is known that the ANXA1 has an anti-inflammatory action due also to the stimulation of the release of anti-inflammatory cytokines [23]. It is therefore conceivable that the ANXA1 exposed on the cell membrane represents an anti-inflammatory signal and contributes to the removal of apoptotic cells without triggering an inflammatory response. Alterations in the expression of ANXA1, increase or decrease, were observed in a wide variety of tumors. Numerous studies conducted over the past decade have revealed an important role dall'ANXA1 in the process of apoptosis and as recognition signal "Eat-me" ("Eat") expressed on the membrane of apoptotic cells [24] .
In this work has been shown that the ANXA1 is a ligand of engulfment transported from the cytosol to the membrane where colocalizes with phosphatidylserine during the final stage of clarification of the apoptotic process. On the basis of these premises, the aim of this work was to study the role of ANXA1 in apoptosis induced by xanthohumol in human glioblastoma cells
In the first phase of this work, we evaluated the apoptosis induced by xanthohumol in different glioblastoma cell lines with different degrees of malignancy: the T98G (WHO IV), the U87-MG (WHO III-IV) and U343-MG (WHO III) cells . The apoptosis was evaluated after treating cells with xanthohumol at different concentrations and different times. The results obtained show that the effects of xanthohumol on apoptosis in T98G cells are already detectable at 24 hours with a maximum effect at 48 hours after treatment at a concentration of 20 uM. Furthermore xanthohumol is capable of inducing a small effect on apoptosis of U87MG cells only at the highest concentration at 48 h, whereas in cells U343MG gives no significant effect to the times and at the concentrations used. These data reveal a specific selective action because the cells of high-grade malignancy T98G cells are much more sensitive U87-MG and U343-MG cells action of xanthohumol. On the basis of these results was chosen as the optimal concentration for use of xanthohumol that of 20 uM. To assess a possible modulation of ANXA1 induced by xanthohumol in the three lines we used the technique of Western blotting and evaluated the expression of the protein at different times. The data obtained show that xanthohumol induces increased expression of ANXA1 only in T98G cells, in fact there is no change in the levels of expression of ANXA1 nor in U87-MG nor in U343-MG cells. The effect of xanthohumol in T98G on the expression of ANXA1 is time-dependent. Xanthohumol induces a significant increase in levels of the protein at 16 hours after stimulation, with a maximum peak of expression at 48 hours. A better understanding of the expression and function of ANXA1 in tumor biology may lead to the identification of new markers of tumor progression and potential targets for therapeutic intervention. To example, the expression of ANXA1 is little present in undifferentiated tumors with a high degree of malignancy [25].
Based on preliminary data obtained about apoptosis and the expression of ANXA1 following treatment with xanthohumol, in different glioblastoma cell lines, the next step was to investigate the role of ANXA1 in apoptosis induced by XH in cells glioblastoma T98G where the expression of protein by the XH was up-regulated. So we have assessed the possible modulation of ANXA1 expression by xanthohumol in the cytosol and on the membrane of cells T98G by Western blotting technique. The data obtained reveal that the effect of xanthohumol on the ANXA1 expression in the cytosol is time-dependent. Xanthohumol induces an increase of the protein, with a peak expression at 48 hours. Furthermore XH is capable of regulating the expression of ANXA1 also on the surface of the cell membrane which increases already at 16 hours after stimulation with a maximum peak of expression at 48 hours. Xanthohumol is then able to promote translocation and thus the exposure of ANXA1 on the membrane of cells T98G. These data have been confirmed by the analysis of confocal fluorescence microscopy which shows a highly significant accumulation of ANXA1 on the cell membrane as a result of treatment with XH at 24 hours compared to control cells where the expression in membrane was hardly visible. The ANXA1 exposed on the membrane of T98G cells induces to hypothesize a possible role of the protein as signal "eat me" on apoptotic cells and therefore an important role in facilitating the elimination of apoptotic cells by macrophages without producing phenomena of inflammatory type. Our current studies are evaluating the role of ANXA1 exposed on the membrane of T98G cells in phagocytosis by macrophage cells human. The study of these mechanisms will clarify the role of the protein in the extracellular level and can open new developments for the study of drugs that target ANXA1 in the mechanisms that facilitate the removal of tumor cells without activating processes of inflammatory type. The presence of annexin A1 is also important to maintain homeostasis of the brain and was also found in microglia where McArthur et al. [26] have suggested that he was involved in the removal of apoptotic neurons in both inflammatory and non-inflammatory conditions.
The next step was to investigate the effects of xanthohumol of ANXA1 at the transcriptional level thus assessing the levels of mRNA for ANXA1. The results of the analysis obtained by RT-PCR shows that XH induces an increase in mRNA levels for ANXA1 in a time-dependent with a peak at 24 hours thus confirming that XH regulates transcriptional expression of the protein. The promoter dell'ANXA1, in fact, has an AP1 site which can be stimulated by phorbol esters and induce the expression of the protein [27]. It is also known that xanthohumol is capable of stimulating the region of the AP-1 promoter ANXA1 thus inducing an increase in the levels of interleukin-2 in T cells [28] At this point to verify the real involvement of ANXA1 in apoptosis induced by XH, in T98G cells, we performed transfection using a small interference RNA directed against ANXA1 to silence the expression of the protein. Following 48 hours of incubation with the siRNAs and following treatment with xanthohumol, the protein extracts were analyzed by western blotting technique. In control cells xanthohumol significantly increases the expression of ANXA1. The cells transfected with siRNAs-scrambled present ANXA1 levels comparable to those of the control cells while in those transfected with siRNAs directed against ANXA1 protein expression was inhibited significantly both in the presence and absence of xanthohumol. Last phase of this paper was to examine whether the levels of ANXA1 could affect the xanthohumol-induced apoptosis in T98G cells. To do this we employed the small interference directed against ANXA1 for the expression, scrambled siRNA control and subsequently evaluated apoptosis induced by xanthohumol.
The data obtained show that, in cells transfected with control siRNA induced apoptosis xanthohumol is almost unchanged compared to the cells not transfected and treated with xanthohumol, whereas in cells transfected with siRNAs anti ANXA1 where the expression of ANXA1 was significantly inhibited the 'apoptosis induced by xanthohumol resulted partially, but significantly inhibited. To confirm the role of ANXA1 in the mechanism of apoptosis we also assessed the activity of caspase-3 after transfection with siRNA-ANXA1 in T98G cells using fluorimetric assay. The results show that the activity of caspase-3 has decreased significantly in cells transfected with siRNA-ANXA1 after 24-48 hours from treatment with xanthohumol compared to treatment with xanthohumol in cells not transfected with xanthohumol and in cells transfected with siRNA-scrambled control. These data demonstrate that in the absence of ANXA1, the apoptosis induced by xanthohumol is significantly reduced confirming the involvement del'ANXA1 in the activation of caspase-3 and in the mechanism of cell death induced by xanthohumol.
CONCLUSION
The results obtained in this work show that:
- Xanthohumol induces apoptosis in T98G glioblastoma multiforme cell lines with a high degree of malignancy. These cells prove to be very sensitive to its action, and do not see any significant effect in other lines of glioblastoma to malignancy grade below which the U87MG and U343MG cells.
- Xanthohumol positively regulates the expression of ANXA1 in T98G cells with a mechanism of transcriptional type (Fig. 7)
Fig. (7).
Xanthohumol positively regulates the expression of ANXA1 in T98G cells with a mechanism of transcriptional type.
- Xanthohumol induces the translocation of ANXA1 from the cytosol to the cell membrane positively adjusting the exposure on the membrane of T98G cells late in the final stage of apoptosis. ANXA1 could then be a signal of phagocytosis to facilitate the removal of dead cells by macrophages.
- ANXA1 is partially involved in the mechanisms of apoptosis induced by xanthohumol of T98G cells by regulating the activity of the protease caspase-3.
- Further studies on the mechanisms mediated by ANXA1 in glioblastoma multiforme may be important for the development of new drugs that regulate the apoptotic process in these malignant disease
CONFLICT OF INTEREST
The authors confirm that this article content has no conflicts of interest.
ACKNOWLEDGMENT
The results of this paper were presented as poster in the 6th European Congress of Pharmacology (EPHAR 2012).
REFERENCES
[PubMed Link]
[PubMed Link]
[PubMed Link]
[PubMed Link]
[PubMed Link]
[PubMed Link]
[PubMed Link]
[PubMed Link]
[PubMed Link] [PMC Link]
[PubMed Link]
[PubMed Link]
[PubMed Link]
[PubMed Link]
[PubMed Link] [PMC Link]
[PubMed Link]