All published articles of this journal are available on ScienceDirect.
Coordination Between Nitric Oxide and Superoxide Anion Radical During Progressive Exercise in Elite Soccer Players
Abstract
Background:
Exercise increases production of reactive oxygen and nitrogen species (RONS) via several mechanisms. Inter alia, increased blood flow during exercise exposes endothelial cells to shear stress, resulting in increased nitric oxide (NO) production. Increased oxygen consumption or hypoxia during exercise induces increased production of superoxide anion radical (O2-).
Objective:
This study investigates the effects of maximal progressive treadmill exercise test on time-course of peripheral blood NO and O2- production, as well as the effect of long-term training on NO bioavailability.
Methods:
Blood samples of 19 elite soccer players were gathered immediately before the test, during last 10 sec of every test stage, and during active recovery phases.
Results:
Significant increase (p<0.05) in NO production (estimated through nitrites (NO2-)), found between stage I (5.69 ± 1.32 nmol/ml) and basal values (5.36 ± 1.25 nmol/ml), was followed by the decrease in stage II (4.21 ± 0.42 nmol/ml) and production lower than basal to the end of the test. Significant increase (p<0.05) in O2- values was found between stage I (4.18 ± 0.77 nmol/ml) and resting values (4.01 ± 0.69 nmol/ml), and at stages V (4.24 ± 0.85 nmol/ml) and 1st phase of recovery (4.39 ± 0.92 nmol/ml).
Conclusion:
The regression lines of NO2- and O2- crossed at the level of anaerobic threshold, suggesting that anaerobic threshold could be of a crucial importance not only in the anaerobic and aerobic metabolism but in mechanisms of signal transductions as well. Long-term exercise increases NO bioavailability, and there is positive correlation between NO bioavailability and maximal oxygen uptake (VO2max).
INTRODUCTION
Since energy demands and oxygen consumption increase several-fold during exercise, production of reactive oxygen and nitrogen species (RONS) also increases, threatening to disturb redox balance and cause oxidative stress. Free radicals are constantly being generated in a small extent in the body even in rest, and since they have a potential to react with a variety of chemical species, they have multiple functions in cell signaling [1, 2] and enzymology [3]. A rise in their production doesn’t have to be considered detrimental, since, in accordance with the principle of hormesis, such an increase appears necessary to allow for an up-regulation in endogenous antioxidant defenses [4, 5]. But, their excessive production or insufficiency of antioxidant system (AOS) may lead to lots of pathological processes and diseases [6, 7].
The mechanisms of exercise-induced oxidative stress are not yet well understood. Since exercise increases body oxygen uptake by as much as 20-fold, and oxygen flux through active muscle may increase by approx. 100-fold above the resting values, it is clear why mitochondria was considered major source of free radicals [8]. However, majority of studies that have shown increase in mitochondrial superoxid anion radical (O2-) production were performed in vitro, i.e. in conditions that are not adequate to the ones in an activated muscle, so recent studies argue against mitochondria as dominant source in exercise-induced production of free radicals [3, 8]. Alternative mechanisms by which exercise promotes free radical production include: 1) the role of xanthine oxidase (XO) in O2- production during ischaemia-reperfusion, 2) neutrophil NADPH oxidase that especially contributes to O2- production during exercise-induced musle damage and inflammation (e.g. delayed onset of muscle soreness), 3) cyclooxygenase (COX) which products O2- while simultaneously generating prostaglandins (PGs), 4) cytochrome P450 (CYP), 5) nitric oxide synthase (NOS), 6) increased secretion and autooxidation of catecholamines, 7) autooxidation of myoglobin and haemoglobin [3, 8-15].
Exercise-induced oxidative stress can elicit both positive and negative effects on the body. Positive effects of long- term training include up-regulation of many cell processes and gene expression [16, 17], which leads towards improvement of sports performance, while negative effects are often connected with overtraining [18]. The extent of oxidative stress induced by acute bout of exercise depends on many factors, inter alia, exercise mode, intensity, and duration, because different modes of exercise differ in energy demands, oxygen consumption and mechanical stress to tissues [4]. The extent of exercise-induced oxidative stress also depends on gender, age, nutrition habits of subjects, antioxidative supplements they take, etc. [19, 20].
The aim of the present study was to assess time-course of plasma nitric oxide (NO) and superoxide (O2-) production during progressive exercise test, as well as to analyze the cause and result of changes in their production in basal conditions, during exercise and recovery, within a group of 19 elite athletes.
MATERIALS AND METHODS
Subjects
The research was carried out within a group of 19 elite soccer players with more than 3 years of training experience in elite competition (74%) (height: 183.74 ± 5.51 cm, weight: 78.82 ± 7.29 kg, age: 22 ± 2.3 years, VO2max: 59.69 ± 4.56 ml/kg/min).
The data on coronary risk factors, symptoms, medications, and prior cardiac events were gathered before an exercise testing, and no contraindications for the stress testing were found.
The study was done in pre-seasonal period in aerobic training regime. Daily data were recorded on the duration and intensities of training activities performed during the study period (90 minutes of training duration once a day with effort corresponding to a zone of 70-80% of maximum predicted heart rate).
All the participants gave a written informed consent.
The study was approved by Ethical committee, School of Medicine, University of Belgrade.
Protocol
The following method for a maximal exercise testing was used in order to investigate a biochemical endothelial response in exercise. Maximal progressive exercise test was performed according to the modified Ellestad Memorial Hospital B protocol [21] on treadmill (T 200, Cosmed, Italy). Modified Ellestad B protocol is presented in Table 1.
Ellestad B protocol - Modified Version
Load | Duration of exercise | Speed km/h | Grade % | |
---|---|---|---|---|
0 | Rest | 0 | 0 | 0 % |
I | Stage I | 180 sec. | 2.7 | 10 % |
II | Stage II | 180 sec. | 4.8 | 10 % |
III | Stage III | 180 sec. | 6.4 | 10 % |
IV | Stage IV | 180 sec. | 8.0 | 10 % |
V | Stage V | Until exhaustion | 8.0 | 15 % |
R1 | Recovery I | 90 sec. | 6.4 | 10 % |
R2 | Recovery II | 90 sec. | 4.8 | 10 % |
Biochemical Assays
The experiment started in the morning (between 8 and 9 AM). A small cathether was inserted into an antecubital vein for venous blood sampling.
Venous blood was drown with a 5-ml syringe and mixed with 100μL of 3M perchloric acid, 400μL of 20mM EDTA in a 10 ml test tube and after incubation in ice for 20 minutes, centrifuged at 15000 rpm for 5 minutes. The samples were than separated and kept at -20°C until measurement. Blood was drawn: 1) before the test, 2) in last 10 sec of each level of the exercise testing (I-V) (without test interrupting), as well as 3) in the 90th and 180th second of the recovery. O2- production was measured by SOD inhabitable conversion of nitro blue tetrazolium (NBT) to formazan [22]. Nitrite (NO2-) level, as a marker of NO metabolism, was measured by Griess reaction [23].
Oxygen Consumption
The pulmonary VO2, CO2 production (VCO2), and the expired minute ventilation (VE) were measured continuously through usage of an automated metabolic cart (Quark b2, Cosmed, Italy). Before each test the ambient conditions were measured, and then the gas analyzer and the flowmeter were calibrated with high precision gases. During the lower and exhaustion load levels the VO2 values were recorded as averages of 15 s. The participants stated their subjective feeling of exhaustion by using Borg’s CR10 exhaustion scale of at least 8 [24]. The heart rate was monitored continuously and recorded as averages of 15 s using a Polar Electro Oy, Finland.
We hypothesized that the maximal oxygen consumption VO2max was reached when the oxygen consumption got its plateau (the time when increasing of workload cannot affect an increase in oxygen consumption) [25].
Statistics
Results are expressed as means ± standard error of the mean. Two-way analysis of variance (ANOVA) was used to assess variations in NO2- and O2- production depending on 1) their basal production and levels of effort, 2) achieved VO2max and levels of effort. Test of signs was used to evaluate the difference of NO2- and O2- concentration in different load levels. Linear regression was applied to identify connectivity between NO2- and O2- concentration during load progression. The significance level was set at P<0.05.
RESULTS AND DISCUSSION
In 1978 Dillard et al. were the first who connected physical activity with free radical production and oxidative stress [26]. Since the early work of Dillard and colleagues, oxidative stress has received considerable attention, with close to 300 published original investigations [4]. However, majority of these studies were performed in vitro, i.e. in conditions that are not adequate to the ones in an activated muscle, so they have to be accepted with limitations. In vivo studies mostly measured free radical production after a bout of exercise, so the real extent of their production during the exercise remained unknown [8]. In our study, blood sampling was done before the exercise test, during every stage of exercise test and after finishing the test, i.e. during the first 3 minutes of active recovery, so we could monitor the time-course of NO and O2- production during the exercise.
Basal NO2- and O2-Production
Mean basal NO2- and O2- values of 19 subjects are shown in Table 2.
Basal Values of NO2- and O2-
Basal value | NO2- (nmol/ml) | O2- (nmol/ml) |
---|---|---|
X ± SD | 20.34 ± 14.01 | 6.56 ± 5.78 |
Basal values of NO2- showed great variation between subjects (mean value 20.34 ± 14.01 nmol/ml), so we tried to assess whether there is a correlation between basal production of NO2- and subjects’ training history. It was shown that the relation exists, i. e. that athletes who have trained professionally more than 3 years without longer cessations in training process (more than 6 weeks) had greater basal production of NO2- than subjects who have trained less or had training cessations. So, we confirmed the assumption that long-term training induces increase in NO bioavailability, whilst training cessations, i. e. physical inactivity, have negative effect – it leads to reversible changes and preclude the increase in NO bioavailability. That is in accordance with studies that showed that physically active people have greater basal NO production than sedentary population [27-29], as well as that exercise induces increase in basal NO production [30-32], especially in sedentary [33] and people with some kind of endothelial dysfunction [34-37]. However, it should be emphasized that these exercise-induced changes in NO bioavailabilty are transitory and can be lost during inactivity period [32].
NO2- and O2- Concentrations During the Exercise Test
As original values of NO2- and O2-had high coefficient of variation, values were exponentially transformed, and transformed values are shown in Table 3. Time-course of NO2- and O2-production during exercise is shown in Fig. (1).
NO2- and O2- Concentrations During the Exercise Test
Load | NO2- nmol/ml X ± SD |
O2- nmol/ml X ± SD |
|
---|---|---|---|
0 | Rest | 5.36 ± 1.25 | 4.01 ± 0.69 |
I | Stage I | 5.69 ± 1.32 | 4.18 ± 0.77 |
II | Stage II | 4.21 ± 0.42 | 4.12 ± 0.65 |
III | Stage III | 4.38 ± 0.51 | 4.14 ± 0.72 |
IV | Stage IV | 3.97 ± 0.31 | 3.99 ± 0.64 |
V | Stage V | 3.98 ± 0.38 | 4.24 ± 0.85 |
R1 | Recovery I | 3.98 ± 0.33 | 4.39 ± 0.92 |
R2 | Recovery II | 4.03 ± 0.49 | 3.93 ± 0.72 |
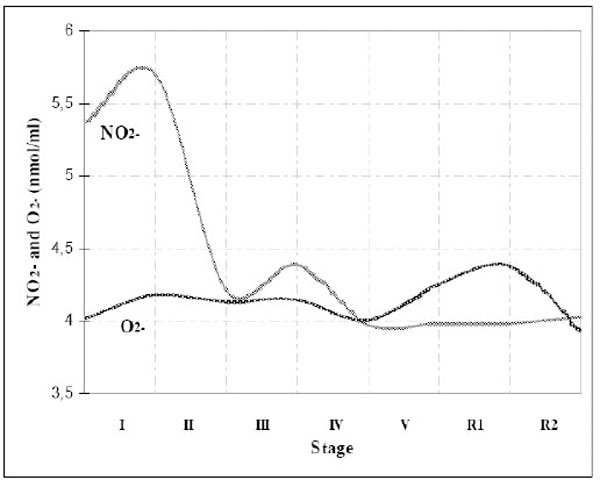
Time-course of NO2- and O2- production during the exercise.
Statistically significant (p<0.05) difference in NO2-production was found between resting state and grade I (increase in NO2-production), and between stage I and stage II (decrease in NO2- production). NO2-stayed decreased (lower than basal) until the end of testing. Increased NO2- production at the beginning of the exercise test can be explained by increased blood flow and shear stress [38]. The subsequent drop in NO2- production may be explained by effects of ROS (O2-) on NO biovailability, since O2- acts as NO scavenger, decreasing his bioavailability [15]. This reaction is especially evident when the levels of NO increase into the elevated nanomolar range and approach the local concentrations of superoxide dismutase (SOD) [39], because the reaction between NO and O2- is 3 times faster than SOD-mediated dismutation of O2-[11, 40]. Later, during the test, the NO level declines, probably as a consequence of a structural adaptation of blood vessel smooth muscle cells in trained subjects [41], the adaptation that subjects in our study gained during long-term training history. Tinken et al. [42] recently confirmed this mechanism of blood vesells adaptation to repetitive exposure to shear stress, emphasizing the time-course of endothelial adaptation - functional changes in the conduit arteries precede structural adaptations in vivo, i.e. NO may play a role in the short-term adaptations to exercise (over a few weeks), but long-term exercise (over a period of years) is associated with another mechanism of endothelial adaptation to increased blood flow [43]. It is possible that adaptations to meet metabolic demands with training evolve from, at least in part, NO-mediated vasodilation in the short-term, to longer term adaptations such as metabolic enzyme changes and vascular restructuring [41-43]. Structural changes (including increased vessel diameter) minimize or even eliminate the need for enhanced release of NO, so it returns to the baseline [42, 43].
Generally, it is thought that exercise increases ROS production, especially intense aerobic exercise during which athletes consume large amounts of oxygen (2-5% of that oxygen, due to electron laekage in mitochondria chain, converts to O2-) [15]. During our testing, statistically significant (p<0.05) increase in O2- production was also found between the resting state and grade I, and also, between the grade IV and V and the first phase of the recovery (R1). The increase of O2- levels with the beginning of the test and latter O2- decrease could be explained by mitochondrial properties to produce free radicals – mitochondrial free radical production increases during state 4 respiration (low VO2; high membrane potential; low ATP production), while during state 3 respiration (high VO2; lower membrane potential; high ATP production) it decreases [44]. That is in accordance with results of Herrero and Barja, who confirmed the decrease of radical production as VO2 and ATP synthesis increase, and observed the lowest O2- concentration at the point of anaerobic threshold [45] (our subjects achieved their anaerobic threshold somewhere near the end of the stage IV). After the point of respiratory compensation (anaerobic threshold), O2- concentration started to increase again, which partly can be explained by U-shaped radical formation related to the mitochondrial pO2 and/or an alternative mechanism – mechanism of xanthine oxidase O2- production during ischaemia and reperfusion [8, 46]. Also, high intensity exercise is often accompanied by increased secretion of the catecholamines which can undergo autooxidation, with and without oxygen, and produce O2- [47].
Time-Course of NO2- and O2- Production During the Exercise - Dependance on their Basal Values
As basal NO2-values of athletes varied to the great extent, we divided them into three groups (depending on their basal NO2-value - B1: NO2-> 20 nmol/ml, B2: NO2-10-20 nmol/ml, B3: NO2-< 10 nmol/ml), in order to detect differencies in time-course of NO2-production between groups, i.e. to see whether basal value of NO2-affects time-course of NO2-production during the test. Analysing the results of these three groups (The two-way ANOVA), we concluded that there were differences in NO2-production between groups (B1-B3 and B2-B3), but that the differencies were not related to the level of effort. The groups producted higher or lower concentrations of NO2-, but the pattern of NO2-production during the test was similar (Fig. 2). All three groups showed initial increase in NO2-production, and the increase was the lowest in the group with the lowest basal NO2-production. After the first level of effort, NO2- production dropped in all three groups, and stayed decreased and relatively stable to the end of the test. The group with the lowest basal NO2-production showed the least oscilations in NO2-production during the test. That is in accordance with the results of the study that compared time-course of NO2-production between athletes from different sports [48]. That study showed statistically significant variations in NO2-production between athletes of different sport, both in basal and active conditions, and also that athletes with the lowest basal NO2-production had the least oscilations in NO2-production during the test.
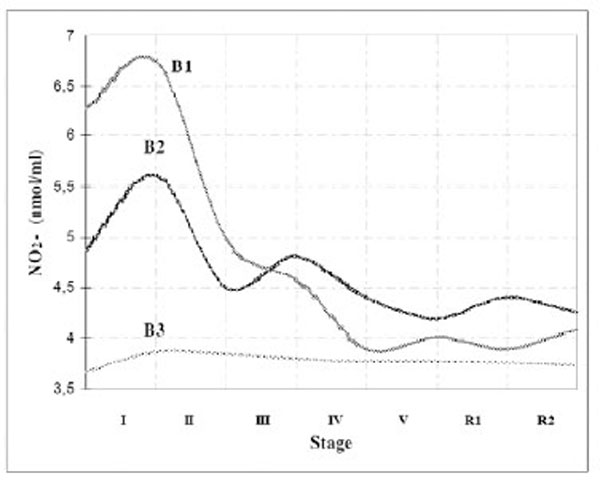
Time-course of NO2- production during the exercise test - dependance on its basal values.
The two-way ANOVA analysis of O2-production during exercise between groups based on basal O2-production (B1: O2-> 10 nmol/ml, B2: O2-= 5-10 nmol/ml and B3: O2-< 5 nmol/ml) showed that there was significant variation in O2- production between groups, but that it wasn’t in a relation with levels of effort (Fig. 3). We concluded that production of O2-is pretty individual and depends on many factors. For example, basal O2-values depend on physical activity during preceding day(s), i. e. exercise-induced muscle damage and inflammation cause delayed free radical production by neutrophil NADPH [3]. Cyclist in study by Cubrilo [49], had higher basal values of O2-than rowers and taekwondo athletes, due to intensive and long trainings before they participated in the study. Some parameters of oxidative stress do not show themselves immediately after exercise, but hours or days later [50].
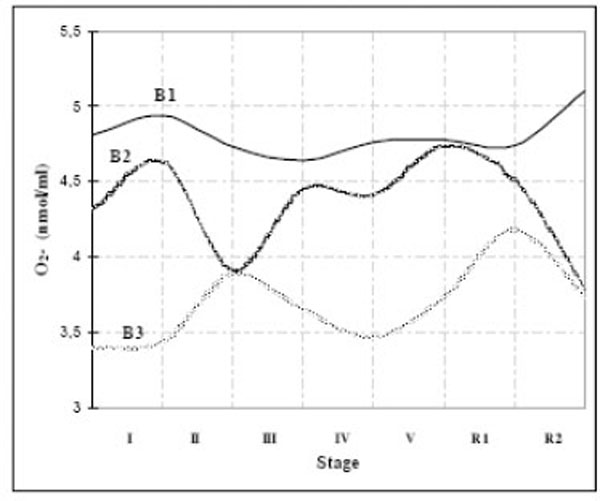
Time-course of O2- production during the exercise test - dependance on its basal values.
Time-Course of NO2- and O2- Production During the Exercise - Dependance on VO2max of Subjects
In order to determine whether dynamics of NO2- and O2- production during the exercise test depends on the level of aerobic capacity of athletes, we divided athletes into three groups, based on the VO2max achieved on the test – G1: VO2max > 65 ml/kg, G2: VO2max = 60-65 ml/kg, and G3: VO2max < 60 ml/kg (Table 4).
Time-Course of NO2- and O2- Production During the Exercise Test – Dependance on VO2max
Stages | 0 | I | II | III | IV | V | R1 | R2 | |
---|---|---|---|---|---|---|---|---|---|
Group | VO2max (ml/kg) | NO2- production (nmol/ml) | |||||||
G1 | > 65 | 5.65 | 6.45 | 4.9 | 4.29 | 3.81 | 3.98 | 3.81 | 3.79 |
G2 | 60-65 | 4.91 | 5.05 | 4.29 | 4.23 | 3.93 | 3.83 | 4.01 | 4.24 |
G3 | < 60 | 5.41 | 5.59 | 4.21 | 4.63 | 4.18 | 4.07 | 4.14 | 4.07 |
Group | VO2max (ml/kg) | O2- production (nmol/ml) | |||||||
G1 | > 65 | 4.32 | 4.66 | 4.48 | 4.56 | 4.39 | 4.53 | 4.93 | 4.02 |
G2 | 60-65 | 3.74 | 3.81 | 3.64 | 3.39 | 3.65 | 3.74 | 4.26 | 3.79 |
G3 | < 60 | 3.74 | 3.87 | 4.15 | 4.03 | 3.72 | 3.95 | 4.17 | 4.06 |
We didn’t find significant variations in time-course of NO2- production between groups, i.e. there wasn’t significant correlation between VO2max and time-course of NO2- production during exercise, but that there was significant correlation between VO2max and NO2- basal production, since athletes who had basal NO2- production > 20 nmol/ml achieved higher VO2max on the test (mean value VO2max = 65,84 ml/kg) compared to athletes who had NO2- basal production < 20 nmol/ml (mean value VO2max = 60,82 ml/kg).
Correlation between NO bioavailability and exercise capacity was also shown in many other studies. Rassaf et al. showed that the capacity of the vasculature to produce NO, and thus nitrite, predicts maximal power and duration of exercise in 55 healthy subjects [51]. Hambrecht et al. suggested that improvement in NO-mediated vascular function improves cardiac function and VO2max in patients with chronic heart failure [36]. Allen et al. also showed significant relation between VO2max and baseline levels of nitrite and nitrate (NOx) [52], but he also found correlation between VO2max and the increase in NO2- or NOx from baseline to recovery [52-54], which is opposite to our results (both nitrite and nitrate increased during exercise in his studies, which is opposite to our results, too).
Analysis of O2- production during exercise showed that there were differencies in time-course of O2- production between groups (G1-G2 and G1-G3) – groups with higher aerobic capacity showed decrease in O2- production after the initial increase in the beginning of the test, while in group with lower VO2max concentrations of O2- continued to rise until the stage III. Trend of decrease in O2- production in groups with high VO2max may be explained by metabolic, cardiovascular, respiratory and endocrine adaptations that developed simultaneously with aerobic capacity development [55]. It was shown that antioxidants appear in blood within less than 5 minutes after occurrence of free radicals [56], i.e during the stage II of the exercise test, so decrease in O2- production may also be explained by activity of antioxidants.
NO and O2- Interaction During the Exercise Test
The reaction between NO (produced as a response of endothelium to increased blood flow and shear stress) and O2- (produced as a result of increased physical activity) is 3 times faster the SOD-catalysed dismutation of superoxide, so when the levels of NO increase into the elevated nanomolar range and approach the local concentrations of SOD, NO is able to compete with SOD for the scavenging of O2-[39]. This interaction results in alleviating O2-toxic effects, but also decreases effects of NO, for example NO-mediated vasodilatation [15].
By following ratio of their production during the exercise test, we detected the point of their most intensive reaction, which turns to be near the grade IV, at the level of anaerobic threshold (Fig. 1). It suggests that lactate threshold could be of a crucial importance not only in anaerobic and aerobic metabolism but in mechanisms of signal transductions as well.
CONCLUSION
There is a growing body of evidence that chronic exercise, via increasing NO bioavailability, expresses beneficial effects on endothelial and cardiac functions. This study adds evidence that long-term training increases basal NO bioavailability, and that there is positive correlation between NO bioavailability and maximal oxygen uptake. It also confirms that these changes can be lost if training cessations occur.
The decrease in NO production during the exercise test found in our study is not in compliance with several other studies that found increased NO production during exercise [27, 51-54, 57], or found that NO didn’t change during or after exercise bout [28, 58], but the differencies are probably due to different protocols, i.e. different characteristics of subjects (age, physical activity, health), training and tests (type, intensity, duration of exercise tests or training), various methods of measuring RONS production…
Novel discovery of this study is that regression lines of NO and O2-production during exercise cross at the point of anaerobic threshold, which suggests that anaerobic threshold is not only of a crucial importance in anaerobic and aerobic metabolism but in mechanisms of signal transductions as well.
General limitation of our study is a small number of participants, but it can be a starting point for further investigation.
ACKNOWLEDGEMENTS
This work was supported by the Grant No. 145014G from the Ministry of Science and Technical Development of the Republic of Serbia.
REFERENCES
[PubMed Link] [PMC Link]
[PubMed Link]
[PubMed Link] [PMC Link]
[PubMed Link]
[PubMed Link] [PMC Link]
[PubMed Link]
[PubMed Link]
[PubMed Link]
[PubMed Link]
[PubMed Link]
[PubMed Link]
[PubMed Link]
[PubMed Link]
[PubMed Link]
[PubMed Link]
[PubMed Link]
[PubMed Link]
[PubMed Link]
[PubMed Link]
[PubMed Link]
[PubMed Link] [PMC Link]
[PubMed Link]
[PubMed Link] [PMC Link]
[PubMed Link]
[PubMed Link]
[PubMed Link]
[PubMed Link] [PMC Link]
[PubMed Link]
[PubMed Link] [PMC Link]
[PubMed Link]
[PubMed Link]
[PubMed Link]
[PubMed Link] [PMC Link]
[PubMed Link]
[PubMed Link]
[PubMed Link]